Study
Objectives
· To define concepts such as cardiac insufficiency, central venous pressure, compliance,
contractility, venous pump, venous return and ventricular stroke work.
· To describe four capacities of the cardiac pump called bathmotropic, chronotropic,
inotropic, and dromotropic states. To describe the pressures of the left and
the right half of the heart. To describe atherosclerosis, arteriosclerosis and risk factors involved.
· To draw pressure-volume curves and contractility lines for the heart at different
conditions, and pressure variations in the left side of the heart and the
aorta.
· To calculate the external work on the blood by the right and left ventricle, and the
kinetic energy.
· To explain the autonomic innervation of the heart, venous return, cardiac contraction,
Starling´s law of the heart, cardiac performance and oxygen demand, and
cardiac filling pressure. To explain ischaemic heart disease, rheumatic heart
disease, Buerger´s disease, and Raynaud´s disease.
· To use the above variables and concepts in problems and case histories.
Principles
· Starling´s law of
the heart: With an increased ventricular filling during diastole (venous
return), the ventricular fibre length increases, so the ventricular
contraction and stroke volume increases. - This is an intrinsic adaptation of
the pumping capacity to the venous return. - Starling’s
law of the heart is also called the Frank-Starling relationship, because it
was described independently by Otto Frank and Starling.
· The
Fick principle (cardiac output) states that the volume of oxygen taken up by
the blood in the lungs, divided by the arteriovenous oxygen content
difference, is equal to the cardiac output. This example utilises the law of
conservation of matter.
· The
law of Laplace - Eq. 8-6
· Poiseuille´s
law - Eq. 8-3.
Definitions
· Angina
pectoris (chest pains) is
pain felt beneath the sternum or in the precordial area. The hypoxic pains are
typically provoked by exercise or cold and submitted by subendocardially
situated nerve fibres. The pains are relieved rapidly by nitro-glycerine and
rest.
· Arteriosclerosis refers to atherosclerosis (and further changes) of the peripheral arteries.
· Atherosclerosis is a process of progressive
lipid accumulation (atheromatosis)
and calcification of the inner arterial walls in the abdominal aorta, lower
extremities and the arteries of the heart, brain and kidneys.
· Bathmotropic
state refers to the
irritability of the myocardium.
· Cardiac
insufficiency is a disorder,
where the heart cannot pump enough blood to satisfy the nutritive needs of the
body.
· Central
venous pressure (CVP) is the
pressure measured in the caval veins at the level of the heart or in the right
atrium.
· Chronotropic
state refers to the cardiac
frequency.
· Compliance of the resting cardiac chambers refers to dV/dP (chamber compliance) - the reverse of the elastance (dP/dV) of relaxed tissue.
· Cardiac
contractility is the dP/dV of the contracting ventricle. The contractility is depicted on the
pressure-volume loop of the cardiac ventricle. Contractility refers to the
change in slope of the pressure and volume increase from isovolumetric rest to
contraction. Contractility is a function of contraction by crossbridge
cycling.
· Ejection
fraction refers to the stroke
volume of blood as a fraction of the end-diastolic ventricular volume. This is
a useful index of contractility.
· Inotropic
state is another term for the
myocardial contractility.
· Intermittent
claudication refers to
chronic ischaemia of the legs with hypoxic pains while walking.
· Dromotropic
state refers to the
conduction velocity of the myocardial conduction system.
· Maximum
oxygen uptake is the oxygen
uptake during maximum exercise. This is a measure of endurance
capacity, and when expressed per kg of body weight it is also called the fitness
number (ml min-1 kg-1).
· Mean
circulatory equilibration pressure (MCEP) is the filling pressure everywhere in the circulatory system following
cardiac arrest.
· Venous
pump refers to all local external forces acting on valvular veins and
facilitating venous return.
· Venous
return is the bloodflow reaching the right atrium (in steady state a similar
bloodflow reaches the left atrium).
· Ventricular
stroke work is the work
applied to the blood at each ejection from the ventricle.
Essentials
This paragraph deals with 1. Cardiac
electro-mechanics, 2. Pressure-volume work, 3. Venous return, 4.
The venous pump, 5. The lipoprotein
metabolism, and 6. Risk factors.
1.
Cardiac electro-mechanics
The heart is a
four-chambered double pump. Every 24 hours the heart is ejecting more than 104 l of blood, and contracting more than 105 times. The total amount
of work performed over the lifetime of a person is enormous. The order of size
is calculated at the end of this chapter.
The cardiac cycle
describes volume, pressure, and electric phenomena in the left ventricle as a
function of time, and one heartbeat is shown below (Fig.
10-1). The red
clock-shaped curve is the intraventricular
pressure. The left ventricle is closed to the aorta in diastole and blood
flows from the atrium to the left ventricle (Fig. 10-1). The ECG is explained
in Chapter 11. Contraction or ventricular
systole results in closure of the mitral valve (Fig. 10-1).
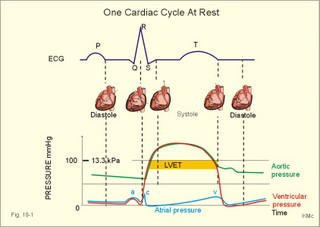
Fig. 10-1: Electro-mechanical events in the cardiac cycle. The aortic
pressure is shown with a green curve. The blue atrial pressure curve has a, c,
and v waves. For the ECG see Chapter 11.
The systole is isovolumetric (ie, the volume of blood in the ventricle is unchanged) until the
intraventricular pressure exceeds the aortic pressure. Then the aortic valve
opens and ventricular ejection occurs (see thick upward arrow in Fig. 10-1). Bulging of the cuspidal mitral valve into the left atrium during isovolumetric
contraction causes a rise in left atrial pressure (see the c-wave in the thin a-c-v-curve of Fig.
10-1). The intraventricular pressure reaches a plateau around 15-16 kPa and
then begins to decrease. The aortic valve closes when the intraventricular
pressure falls below aortic pressure (see small arrow indicating retrograde flow in aorta
in Fig. 10-1). This is the end of the ejection phase or the left ventricular ejection time (LVET).
The electrocardiogram (ECG) does not reflect the mechanical performance
of the heart, but closure of the
aortic valve corresponds in time with the end
of the T-wave in ECG (Chapter 11).
The a-wave occurs during the contraction of the right atrium by which it squeezes out extra blood just before ventricular systole
(early atrial contraction). As the atrium relaxes, the CVP is reduced (blue
atrial curve in Fig. 10-1). The next atrial wave is the c-wave, and this wave is produced by closure of the cuspidal valves and by the right ventricular contraction, because the increased
ventricular pressure is transmitted backwards to the right atrium and large
veins.
Filling
of the atrial chambers with blood is aided by the large atrial compliance. This
is why the atrial pressure only rises modestly. The third atrial wave is the v-wave for venous return. Throughout ventricular systole and isovolumetric
relaxation, venous blood returns to
the heart, but the tricuspid valve is closed, so that the central veins and
right atrium are distended. The coinciding pressure build-up is relieved, when
the tricuspid valve opens at the start of diastole, and the pressure is
reduced (Fig. 10-1). The increase in right
atrial pressure is transmitted backwards to the large veins near the
heart. Prominent waves can often be seen in the neck veins when supine.
The periods just
described for the left heart can be shown to be the same in the right heart,
except for the fact that the systolic pressures are considerably lower in the
right ventricle and pulmonary artery. The stroke volumes of the two ventricles
are the same. Contraction of the right ventricle begins just after that of the
left side and lasts for a shorter time (the peak pressures obtained are much
less).
2.
Pressure- volume work
At the
end of the ventricular diastole the left atrial pressure increases, because
the atrial systole begins just before the ventricular systole.
The intraventricular pressure-volume loop is a
time-independent representation of the cardiac cycle, where the instantaneous
intraventricular pressure and volume is plotted (Fig.
10-2). In diastole from
B to C, the ventricle receives blood from the left atrium. The small increase
in ventricular pressure reflects passive expansion and elastance of the
myocardial wall. Pressure and volume increase with a slope that is related to contractility.
The green line represents minimal contractility. The line starts from the
ventricular dead volume, that is a virtual minimal volume of blood, which can never be
ejected (50 ml in Fig. 10-2). A steep rise in pressure occurs from C to D,
with no change in ventricular volume (the isovolumetric contraction). At D the
aortic orifice opens, because the end-diastolic pressure in the aorta is
passed. During the rapid ejection phase, the fall in ventricular blood volume,
is accompanied by a continuous increase in pressure. During ejection the
volume falls by a size equal to stroke volume, pressure rises and falls until
the residual ventricular volume is attained (about 80 ml in Fig. 10-2). The last
ejection phase is slow, because the pressure decreases towards A, where the
aortic orifice closes. The last event from A to B is the isovolumetric
relaxation with a sharp drop in pressure at constant volume (Fig. 10-2).
The steep slope of the curve refers to optimal contractility at a given
condition (Fig. 10-2). Actually, the precise contractility concept is the change in slope from isovolumetric rest to the highest slope of the curve.
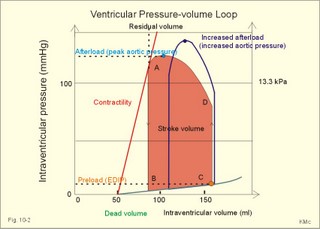
Fig.
10-2: The left ventricular pressure-volume loop from a healthy person at rest. – The pressure-volume loop is a
widely applicable pathophysiological tool.
The area of the loop represents the pressure-volume work on the stroke
blood volume performed by the ventricular contractile elements during
ejection.
The pressure in C is the end-diastolic intraventricular pressure or the so-called preload.
The force against which the ventricle contract is termed afterload. A good index of the afterload is the peak
aortic (or intraventricular) pressure
during systole, equal to the highest pressure shown in Fig. 10-2. The
afterload is almost equal to the peak systolic
pressure in the arterial tree. When the afterload is increased at constant
end-diastolic pressure and volume, a greater ventricular pressure develops in
order to expel blood (the dashed curve from D in Fig. 10-2). The result is a
smaller stroke volume (and hence a greater residual ventricular volume),
because of the high aortic and intraventricular pressure (Fig. 10-2).
The
Frank-Starling relationship states that increasing left ventricular
end-diastolic volume increases the stroke volume of the next heart beat.
During isovolumetric contraction, the end-diastolic intraventricular pressure (EDIP) or preload increases.
Increasing end-diastolic volume increases the ventricular filling pressure and
thus stroke volume and stroke work. An increase in afterload occurs when the
aortic pressure increases. Such an increase causes a decrease in stroke
volume. Hereby, the EDIP and the end-diastolic volume increase, so the cardiac stroke work increases concomitantly
(Fig. 10-2). The ventricular stroke work is the sum of the pressure-volume
work and the kinetic work and
calculated according to Eq. 10-1. Preload is the end-diastolic filling pressure of the ventricle just before contraction
(C and E in Fig. 10-3).
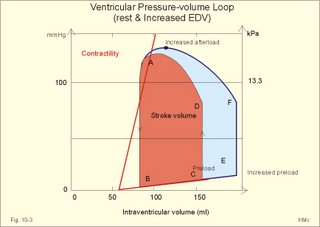
Fig. 10-3: Left ventricular pressure-volume loops at rest (red area) and
following an increase in end-diastolic volume by increased diastolic filling
(blue loop with E-F).
When
the left ventricle expands from C to E, by receiving more blood than before,
the end-diastolic volume is increased. The greater diastolic filling results
in a larger stroke volume according to Starling’s
law of the heart. The Frank-Starling
relationship can be formulated as follows: Any increase of preload
(ventricular filling) invokes a progressive increase in the blood volume
ejected by the ventricles beat-by-beat until the cardiac output equals the
input.The ventricular pressure increases with the rise in systolic aortic
pressure (increased afterload in
Fig. 10-3). The stroke work of the heart on the blood is the area A, B, E, F,
A. Accordingly, the stroke work is increased. A sympathetically mediated
increase in contractility without a change in end-diastolic pressure (preload)
results in an increased intraventricular pressure (Fig.
10-4). The slope of the line through G illustrates the increased contractility. The larger stroke volume, smaller residual
volume, and larger stroke work on the blood (area C, D, G, H) is also shown in
Fig. 10-4.
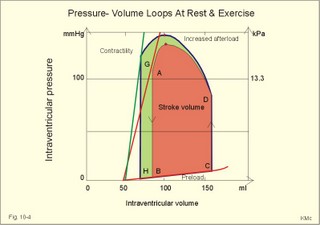
Fig.
10-4: Left ventricular pressure-volume loops from a healthy person at rest and
following an increase in contractility during exercise (G-H).
The
transmural pressure rises during ventricular contraction even at constant
fibre tension. This is because the short ventricular radius is reduced to the
same extent (see the law of Laplace, Eq.
8-5).
Echocardiography shows that the short ventricular radius is reduced by 5-6 mm during systole in
healthy people at rest. The Laplace law is acceptable in such a situation.
If
a cardiac chamber of a heart patient increase its diameter to double, and a
spherical chamber is assumed, it implies a two-fold greater fibre tension (Fig. 8-9B). To the patient, this means an enormous
energy requirement in the myocardium to maintain the necessary pressure. This
disorder is called cardiac failure or
insufficiency (see later).
3.
Venous return
Veins
are highly distensible or compliant vessels
(ie, they have a large volume/pressure ratio, dV/dP) that have one-way valves. The venous system (veins, venules, and venous sinuses)
controls the amount of blood that is translocated from the venous to the
arterial side of the circulation.
In
this paragraph the circulatory system is simplified to a venous system
connected by a heart pump to an arterial system (Fig.
10-5A). The central
venous pressure (CVP) is the pressure measured in the caval veins at the
level of the heart or in the right atrium.
When
the heart pump stops, the pressure is the same in all compartments of the
circulatory system, (ie, the mean circulatory equilibration pressure, MCEP).
MCEP depends upon the blood volume and the compliance of the vessels (Fig.
10-5A). As the heart pump starts and moves blood from the venous system, the
pressure here will fall and the arterial pressure will rise. A further rise in
pumping activity (cardiac output) reduces the central venous pressure (CVP),
and finally the CVP is negative, so the central vessels collapse. This impedes
the bloodflow into the atrium (the venous return), so that the cardiac output
cannot rise any longer (Fig. 10-5A).
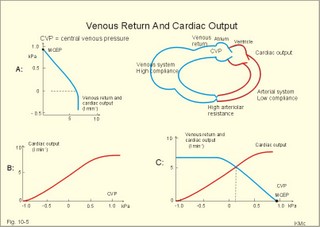
Fig. 10-5: The central venous pressure (CVP) as a function of cardiac
output (A). – The cardiac output as a function of CVP (B). – Combined venous return and cardiac output curves as a function of
CVP (C).
The cardiac output must
be a function of CVP at a given steady state. Increasing the venous return
will increase CVP from –1 kPa towards zero, and increase cardiac output as
well (Fig. 10-5B). As CVP becomes
increasingly positive, it exerts a backpressure on the venous system to impede
venous return. The rise in cardiac output levels off, and there is no further
rise at values around MCEP.
The third step is to
combine the curves in Fig. 10-5 A and B. At the normal CVP (or right atrial
pressure) the venous return curve crosses the cardiac output curve, and both
flows are 5 l per min. If the
atrial pressure is suddenly increased to the mean
circulatory equilibration pressure, then all flow of blood is stopped
(Fig. 10-5C). The low pressure during arrested circulation is mainly due to
the very distensible venous system. The right atrial pressure has only
increased slightly, but enough to decrease the venous return to zero and thus
the cardiac output is zero (Fig. 10-5C). The more the atrial pressure falls
below the venous pressure, the more the venous return will rise up to a
certain level at an atrial pressure of -0.2 kPa (almost zero). Negative atrial
pressures have the same venous return. This is because negative transmural
pressures in the central, thoracic veins imply collapse. The venous return is
therefore constant, regardless of a further fall in right atrial pressure (Fig.
10-5C).
The cardiac output must
equal venous return in the steady state. Thus the cardiac output- curve for
the left ventricle must cross the venous return curve in one point (Fig.
10-5).
The steep part of the
cardiac output curve shows that the cardiac output can double following a
small rise in pressure.
The driving pressure for the systemic
circulation is the mean aortic pressure (MAP) minus CVP. The relationship to
cardiac output and total peripheral vascular resistance (TPVR)
is given by Eq. 10-2. A small fraction of TPVR is found in the venous system.
Eq 10-3 expresses the
venous return. Small variations in CVP alter the volume of blood considerably
in the venous system. A normal value for venule pressure is 1.3 kPa (10 mmHg)
and for CVP about zero. Since venous return must equal cardiac output in
steady state, the venous resistance is only about 10 % of TPVR.
4.
The venous pump
The venous pump is
defined as all local external forces that facilitate venous return to the
heart. Two important pump mechanisms are involved:
1. The
skeletal muscle- pump. The deep veins of the arms and legs are affected by
pressure exerted by exercising skeletal muscles. The veins are compressed by
muscle contraction, and the one-way valves prevent the blood from flowing
backward, and thus secure the transfer of blood toward the heart. Even the
superficial veins are compressed during contraction.
As soon as a venous
segment is emptied of blood, its transmural pressure is so low that the filling pressure from more peripheral veins can fill the empty segment with
blood.
The skeletal muscular
venous pump is also called the peripheral
venous heart. In the erect
position the peripheral venous heart must overcome the force of gravity and
prevent overpressure in the dependent limb. During muscle rest there is an
added hydrostatic pressure load of 13.3 kPa (100 mmHg) in the dependent limb.
With a MAP of also 13.3 kPa the total pressure in a foot artery is 26.6 kPa,
and in the dependent vein just above 13.3 kPa. During muscle contraction the
venous pressure rises driving blood into the heart and just after muscle
contraction the venous pressure falls again.
2. The
thoraco-abdominal pump. The large veins are also affected by the positive
intra-abdominal pressure and by the negative pressure in the thoracic cavity.
The inferior caval vein returns blood from lower regions to the heart. During inspiration the intrathoracic pressure becomes more negative, and blood is sucked into the caval veins facilitating venous return to the heart. The
inspiratory contraction of the diaphragm increases abdominal pressure
favouring venous return. During expiration the intra-abdominal pressure decreases and intrathoracic pressure
increases but remains negative, so that the venous return is maintained. Intrinsic cardiac mechanisms, including the length-tension relation (Chapter 2), allow the heart to increase
stroke volume beat-by-beat, when the
venous return increases. – Straining against a closed glottis is called
Valsalva´s manoeuvre, and it is part of our every day life during coughing,
defaecation, urination and lifting of heavy weights. The intrathoracic
pressure increases abruptly, whereby the venous return is inhibited and the
cardiac output is reduced (Starling´s law). Normally, fainting is prevented
by a strong arteriolar and venous constriction released by the baroreceptor
reflex.
5.
The lipoprotein metabolism
This paragraph is inserted here in order to
help the reader understand the pathophysiology of the most common
cardiovascular disorders.
Lipoprotein particles are build up by a non-polar core containing triglycerides (TG) and cholesterol
esters (E in Fig. 10-6). The polar shell of each particle consists of
phospholipids, apoproteins and non-esterified cholesterol. These substances
provide the particle with a negative electrical charge, which allow it to
remain soluble in plasma (Fig. 10-6 right).
Hepatic synthesis of
cholesterol varies inversely with the dietary intake.
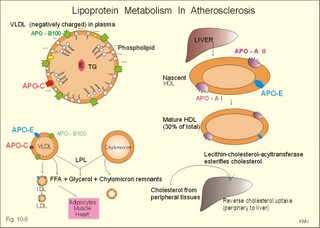
Fig. 10-6: Lipoprotein metabolism (left). - The lipoprotein particles are
found both in the plasma of healthy persons and of patients with
atherosclerosis (right).
Four different lipoprotein particles (VLDL,
IDL, LDL and HDL) control the transport of cholesterol to the cells.
a)
VLDL, IDL and LDLVery low-density
lipoproteins (VLDLs), which
contain mainly the liver-produced TG, are synthesised in the liver and carries
a characteristic surface protein called apoproteins B-100 (Fig. 10-6 right).
VLDL is liberated from the liver in the postabsorptive phase
Chylomicrons are formed
in the enterocyte from dietary fat after a meal and absorbed from the
intestine into the blood (Fig. 22-13). Cylomicrons contain all the dietary
lipids including lipophilic vitamins and have a half-life of 5 min.
VLDL from the liver, and
chylomicrons absorbed from the intestine, are hydrolysed by the enzyme lipoprotein lipase (LPL) on the endothelial surfaces in
the capillaries into glycerol, chylomicron remnants and free fatty acids
(FFAs). From the FFAs the TG molecules are resynthesised and used or stored in
adipocytes, heart and striated muscle cells (Fig. 10-6).
As more and more TG is
removed from VLDL, the density of the particles becomes greater, and they are
now termed intermediate density lipoproteins (IDLs). Normally, the liver cells take up half of
the IDL particles, because they have receptors for the apoprotein B-100 on the
IDL surface. The hepatic lipase removes TG from the IDLs to produce low-density lipoproteins (LDLs) still maintaining their apoprotein B-100. This
apoprotein is recognised by the LDL receptors of all cells. LDL is the largest
cholesterol fraction in blood plasma, and has a half-life of 24 hours. LDL
delivers cholesterol and other lipids from the liver to the cells for
metabolic and structural purposes (forward transport). An increasing
concentration of cholesterol inside the cell automatically down-regulates LDL
receptors and thus regulates the receptor-mediated endocytosis of additional
LDL. The circulating LDL concentration is controlled by the number of hepatic
LDL receptors and by enzyme activity in the cholesterol synthetic pathway.
Genetic LDL receptor deficiency elevates the ratio of LDL to HDL in blood plasma, and a
ratio greater than 4 is a serious risk factor for cardiovascular disease.
b)
HDLThe remains of the
chylomicrons co-operate with IDL and high-density lipoproteins (HDLs) to form cholesterol esters. Cholesterol esters are
then exchanged for TG in VLDL and chylomicrons by the cholesterol
ester-transfer protein, whereby HDL3 changes to the less dense
HDL2.
HDL is the substrate for lecithin-cholesterol
acyltransferase (LCAT). This enzyme catalyses the conversion of free
cholesterol to cholesterol ester. LCAT is reduced in severe liver disease.
HDLs in plasma are disk
formed particles, mainly produced in the liver. They contain an entirely
different apoprotein called apoprotein Apo-I or Apo-II, and also Apo-E (Fig.
10-6 right). In the fasting state, the HDL concentration in the blood plasma
is generally increased in females, by oestrogens, by exercise, and by moderate
alcohol intake.
Similarly, fasting HDL
concentrations are reduced (and LDL increased) in males, by androgens, by
smoking, by obesity, and by an inactive sedentary life-style.
The cell membranes
contain specific HDL receptors. HDL absorbs cholesterol in peripheral tissues
and thereby matures from the nascent state (Fig. 10-6 right). LCAT is
activated by the apoprotein A on the HDL surface. Mature HDL facilitates the
transport of cholesterol back to the liver ((backward transport or reverse
cholesterol uptake), where it binds to Apo-E.
Normally, HDL particles
carry 30% of the total quantity of cholesterol in the blood. HDL protects
against development of atherosclerosis, and a high HDL/LDL ratio reduces the
risk of cardiovascular disease.
Population groups at risk
are advised to eat a low fat diet with unsaturated lipids and a low
cholesterol content, in order to prevent or delay the development of
atherosclerosis.
Persons with extremely
low total cholesterol in their plasma demonstrate a higher mortality than
persons with values around 5 mM. The reason for the increased mortality
(mainly death of cancer and gastrointestinal diseases), is probably
insufficient immune defence and genetic factors.
6.
Risk factors
A risk factor for a disease is a factor showing a statistical
co-variance with the disease. A risk factor is not identical with a definite disease
factor, where all causal steps are clearly understood. Nevertheless, risk
factors may obtain an increasing degree of causal relationship to the disease.
Two or more risk factors present frequently potentiates the risk.
Major risk factors that
predispose to atherosclerosis and ischaemic heart disease (IHD is
atherosclerosis if the coronary arteries) are consequences of the Western
World lifestyle. These consequences are often notified as age changes. The
fact remains that the western life style is characterised by unhealthy
fast-food, a high dietary fat fraction, obesity, years with lack of exercise,
low fitness, smoking, hypertension, hyperlipidaemia, diabetes, gout, oral
contraceptives (synthetic steroids), drugs and doping (testosterone or other
steroids in excess).
Consideration of risk
factors must be supplied with other relevant information in order to provide a
whole patient status, including genetic and immunological factors as mentioned
above.
The inactive lifestyle of
the Western World is documented by measurement of a low maximal oxygen uptake
(endurance capacity) as an average for large population groups. Values below
34 ml per kg and per min are unhealthy for any age. Such a low endurance
capacity is related to high mortality (Chapter
18), especially from IHD (males
and postmenopausal females). Regular exercise seems to protect against IHD.
The following risk
factors for IHD are dealt with below: Obesity, male sex, smoking,
hyperlipidaemia, familial hypercholesterolaemia, diabetes mellitus, gout and
asymptomatic hyperuricaemia, oral contraceptives or synthetic steroids for
doping.
Obesity is clearly
associated with IHD, but probably not linked independently to IHD. The obese
patient is characterised by an inactive lifestyle, low fitness, and preferring
a fatty diet.
Sex. Males are more
frequently affected by coronary artery disease (IHD) than fertile females. The
smaller incidence in females is obviously related to the presence of natural
female sex hormones (oestradiol in natural dosage). After the menopause, the
female incidence approaches that of males. Female sex hormones in natural
dosage may be protective, and male sex hormones atherogenic. Testosterone stimulates hepatic cholesterol synthesis.
Smoking a certain number
of cigarettes per day is directly related to the incidence if IHD, and
following 10 years of abstention, the risk declines towards the normal level.
Hypertension is
associated with an increased risk of IHD (Chapter
9). Most forms of
hypotensive therapy only reduce the risk of cardiac events to some extent, but
clearly reduce the risk of stroke.
Some hypotensive drugs reduce the arterial blood pressure but still have
unwanted side effects. Light exercise reduces the blood pressure and implies
other beneficial effects.
Hyperlipidaemia. Total
cholesterol, HDL with calculation of LDL, and triglyceride
concentrations
should be measured in all patients. High total serum cholesterol combined with
a low HDL, and also high triglycerides are associated with an increased risk
of IHD (Fig. 10-6).
Heterozygous familial hypercholesterolaemia is a relatively common genetic defect caused by mutations in the gene
coding for the LDL receptor. With defective genes there is malproduction of LDL receptors in the liver. Some patients are without physical signs
– others have cholesterol deposition around the eyes (xanthelasma) or in the
tendons (xanthomas). These patients require diet with fibres and reduction of
the cholesterol intake. Alcohol consumption must be reduced. The body weight
must be kept close to ideal with exercise. Usually the patients require
treatment with lipid-lowering drugs.
Homozygous familial hypercholesterolaemia is extremely rare. These patients are without LDL receptors in the liver, so
they accumulate cholesterol and other lipids in the aorta, arteries, organs
and skin. The HDL/LDL ratio in blood plasma is greatly reduced (below 1:4), and the fasting total cholesterol increases towards 30 mM.
Drugs are needed, but the patients usually die young from ischaemic heart
disease.
Diabetes mellitus.
Increased blood glucose after fasting and an abnormal glucose tolerance test is associated with
increased risk of atherosclerosis and a high LDL (Chapter 27).
Gout and asymptomatic hyperuricaemia (Chapter 20) is associated with
an increased risk of ischaemic heart disease and atherosclerosis.
Intake of several types
of oral contraceptives or synthetic steroids for doping (Chapter
18) increases
the risk of atherosclerosis and thrombo-embolic phenomena.
Pathophysiology
This paragraph deals with
heart disorders and atherosclerosis, which cause most people of the Western
Hemisphere to die. Coronary or Ischaemic
Heart Disease (CHD or IHD) is the most widespread. The remaining cases are
caused by arteriosclerotic damage of the brain (strokes) and other organs
(liver, kidney etc).
The two typical
cardiovascular disorders are I.
Atherosclerosis and II. Rheumatic heart disease. - Atherosclerosis is involved
in several widespread disorders (ischaemic heart disease, peripheral arterial disease and hypertension). These diseases are
related to the typical life style of the Western Hemisphere, whereas rheumatic
heart disease is more frequent in poor countries with high frequency of
infections and malnutrition.
I.
Atherosclerosis
Atherosclerosis is a
process of progressive lipid accumulation (atheromatosis)
and calcification of the inner arterial walls in the abdominal aorta, lower
extremities and the arteries of the heart, brain and kidneys.
Atherosclerotic plaques
contain cholesterol, and the most important single factor for their
development is a high plasma concentration of total cholesterol, in particular
a high concentration of LDL.
Atheromas are yellow
streaks or lesions found in arteries at autopsy. They are formed in the intima
(lamina intima) by lipid accumulation in macrophages and monocyte adhesion. As
more and more cholesterol crystals are deposited, the atheromas grow and the
surrounding fibrous and smooth muscle tissue is involved. Finally – as the
subendothelial distortion leads to platelet aggregation - large
arteriosclerotic plaques are formed. They consist of cholesterol and other
lipids, dead cells, collagenous fibres, and there is excessive proliferation
of the smooth muscle cells. The fibrosis or sclerosis makes the arterial wall
stiff, which lead to systolic hypertension. Later Ca2+ salts
precipitate and a factual calcification of the arterial wall may occur.
Typical for
atherosclerosis patients are a high total cholesterol concentration in the
blood plasma (total cholesterol above 6.2 mM), a dangerously high LDL and a
low HDL fraction in fasting plasma (below 20% of the total). Often the
atherosclerotic patient also has a high total triglyceride concentration
(above 2 mM). In a fasted patient the triglyceride concentration depicts the
precursor concentration of dangerous cholesterol: Very Low Density Lipoprotein
(VLDL).
Large atherosclerotic
plaques narrow the arterial lumen and produce arterial stenosis with reduced
bloodflow. Insufficient oxygen delivery to the tissue is called ischaemic
hypoxia, and hypoxic pains develop as in angina pectoris and intermittent
claudication. Total occlusion of the arterial lumen is caused by a thrombus or
an embolus in the lumen, or by wall bleeding. Disruption of the endothelium
results in accumulation of thrombocytes and fibrin with thrombus formation and
a complex atheromatous lesion is produced.
Arteriosclerosis (atherosclerosis) manifests itself in the coronary arteries as Ia. ischaemic
heart disease and in peripheral arteries as Ib. peripheral arteriosclerosis.
Ia. Ischaemic heart disease (IHD)
Atherosclerotic coronary
artery disease remains a leading cause of death, and is manifested as focal
narrowing in the epicardial coronary arteries. The gradually narrowed vessel
segment can be abruptly occluded by clot formation (thrombus) or by
vasoconstriction at the atherosclerotic lesion. When a thrombus flows along
the arterial tree with the blood and occludes the vessel, it is called an
embolus.
Ischaemic heart disease
is caused by reduced bloodflow to a region of the myocardium. Myocardial
ischaemia diminish delivery of oxygen and nutrients, and accumulate
potentially toxic substances such as lactic acid and K+ around the
cardiac cells, whereby necrosis may result. The causes are atherosclerosis with atheromas, thrombosis, emboli, or spasms in the
coronary arteries.
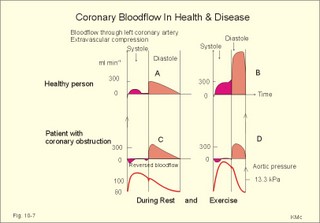
Fig.
10-7: Bloodflow through the left coronary artery at rest and during exercise
in a healthy person (upper) and in a patient with coronary obstruction (lower)
and angina pectoris.
Coronary bloodflow is
restricted in the systole by strong myocardial contractions and in diastole by
the high heart rate of exercise. Normally, the coronary bloodflow in healthy
persons is small during systole and increases during diastole (Fig. 10-7A).
The high heart rate at exercise implies a short diastolic duration, but the
rise in pressure secures a great diastolic bloodflow (Fig. 10-7B).
Four clinical
manifestations of IHD are treated below:
1) Angina pectoris (chest pains) is pain felt beneath the sternum or in the precordial area - often
referred to the left arm-shoulder-neck-jaw etc. Exercise and cold bring on
hypoxia pains in the substernal or precordial area. Hypoxic pains are
transmitted by subendocardially situated nerve fibres. The coronary resistance
vessels contain a-adrenergic
constrictor receptors and badrenergic
dilatator receptors. The vasodilatatory capacity of the coronary resistance
vessels can be maximally mobilised already at rest (Fig.
10-7C). Exercise
shortens the diastolic duration and restricts the rise in diastolic bloodflow
further (Fig. 10-7D). The aggravated myocardial ischaemia results in a lactate
acidosis.
Following sublingual
administration of nitro-glycerine, peak concentrations are achieved in the
plasma within 1 min. Organic nitrates dilatate constricted coronary vessels,
improve the bloodflow to the subendocardial (pain sensitive) part of the
myocardium, and dilatate resistance & capacitance vessels. This dilatation
reduces the venous return to the heart and the arterial pressure (reduced
preload and afterload).
The beneficial effect of
drugs such as glycerol- trinitrate in angina have been known for more than a
century. Recently it was realised that the drugs act by releasing nitric oxide
(NO) in the vascular wall (see Chapter 5).
Ca2+-channel
blockers block the Ca2+ flux into the smooth muscle cells of the
coronary arteries, so they relax. The Ca2+ -channel blockers also
reduce the force of contraction and thus the oxygen demand of the myocardium.
Coronary angioplasty is
a method by which atheromatous obstructions are dilatated by an inflated
balloon.
The arterial oxygen
concentration is also reduced in anaemia, CO poisoning and in shock. Patients
with hyperthyroidism or hypertension may have increased coronary oxygen demand
and all these paitients may experience chest
pains caused by myocardial hypoxia.
Another manifestation of
ischaemic heart disease is myocardial infarction.
2) Acute myocardial infarction (AMI) is due to a sudden coronary thrombosis from an atheromatous plaque
causing cellular death (infarct) of
a myocardial area. Distal to the coronary occlusion the blood pressure is low.
The thin-walled subendocardial vessels are squeezed most and receive the
smallest bloodflow, often leading to subendocardial infarcts. The myocardial infarcts are sometimes silent (which means without pains; the
pain relief is due to destruction of subendocardial nerve fibres). The typical
infarct causes severe and long lasting pain.
Acute myocardial
infarction renders the heart incapable of pumping the minimal blood volume
required to transfer sufficient oxygen to the mitochondria.
The patient experiences a
sudden chest pain and the pain is lasting for hours in contrast to angina.
The patient may develop signs of shock. Necrotic myocardial cells liberate
cellular enzymes such as creatine kinase,
which peaks in the blood plasma within 24 hours. The total enzyme release
depicts the size of the infarction. Lactic dehydrogenase (LDH) isoenzymes
peaks a few days later, and LDH 1 is
rather specific for myocardial necrosis.
Read Chapter 11 before this paragraph:
Non-Q wave infarction:
When only part of the wall is necrotic there are deeply inverted, symmetrical
T-waves (coronary T- waves) and
mostly ST depression in the ECG. These signs of ischaemia are often transient
- only during the acute attack - and found in all precordial leads located
above the infarcted area. Such a subendocardial infarct does not show deep Q
waves, and epicardial involvement implies ST segment elevation.
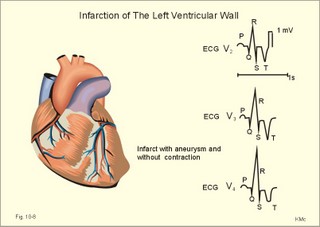
Fig.
10-8: Myocardial infarction of the left ventricular wall with lack of movement
of infarcted tissue during systole. The ECG changes are typical for the
anterolateral location of the infarct (see Ch. 11).
Q-wave infarction: A
wide and deep Q wave in the ECG is a lesion wave, and the sign of transmural myocardial infarction with necrosis through the whole of the
myocardial wall. The deep Q wave is maintained for years after the event. A
typical anterior wall infarction shows changes in lead I and in V2-V6 dependent upon the localisation (Fig. 10-8). During systole the infarcted area
does not contract or move due to cell necrosis (paradoxical movement). There
is always a danger of rupture of necrotic tissue.
A typical posterior
wall infarction is diagnosed by a mirror
image with changes in V1-V2 (reciprocal changes) and ST- depression in lead I. ST-depression
or ST-elevation is indicative of myocardial ischaemia.
Chapter 11 is a prerequisite for the understanding of the above
paragraph!
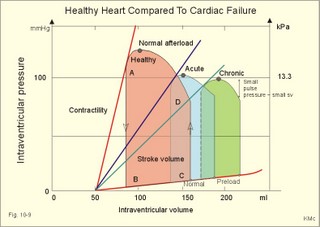
Fig. 10-9: Left ventricular pressure-volume loops in a healthy person
(red) and for persons with acute (blue area) or chronic, congestive (green
area) cardiac failure.
The AMI patient is
extremely tired. Even the work of breathing is a heavy task. The condition is
often a case of general ischaemic hypoxia (Fig. 10-9) and can develop into
cardiogenic shock (see below).
Interaction of platelets
with collagen in the vessel wall is the first step in platelet aggregation
leading to thrombosis. The activated platelets release thromboxanes A2 (TxA2 )
from arachidonic acid in the phospholipids of the platelet membrane. Platelet
aggregation is inhibited by cAMP and by acetyl-salicylic acid, which inhibits
platelet cyclo-oxygenase.
Eicosapentaenoic acid
(EPA) in the diet reduces the frequency of thrombosis by reduction of the TxA2
production.
3) Cardiac failure or cardiac
insufficiency is a disorder, where the heart cannot pump enough blood to
satisfy the nutritive needs of the body. Cardiac insufficiency is manifest by
a consequential decrease in cardiac output (lower
output failure) or by an increase in cardiac output (higher
output failure). The cardiac failure can be acute or chronic.
Damming of blood in the
vessels behind the insufficient heart pump is typical.
Acute cardiac failure is
caused by AMI, acute intoxications, anaesthesia etc. Occlusion of the coronary
artery to the left ventricle impairs contractility, and left ventricular
failure develops due to the diminished cardiac output from the left ventricle.
Initially, the right ventricular output is maintained, whereby the left atrial
pressure (and pulmonary venous pressure) is increased beat-by-beat. As a
consequence, the left ventricular output will increase until the cardiac
outputs of the two ventricles are equal. The increased pulmonary venous
pressure leads to reduced lung compliance (dV/dP) and increased respiratory
elastic resistance with increased respiratory work and distress. Eventually,
plasma fluid flows into the alveoli and pulmonary
oedema is developed (Fig. 10-10).
Chronic or congestive cardiac failure occurs in conditions such as IHD and following severe hypertension. In chronic
cardiac failure blood is accumulated and expands the venous system and the
left ventricle (Fig. 10-9).
Cardiac oedema develops
during congestive cardiac failure, because the kidneys retain NaCl and water.
The accumulated fluid increases venous return, which in turn elevates the
right atrial pressure. The rising atrial pressure elevates the venous and the
capillary pressure. This causes loss of fluid into the interstitial fluid
volume. Accumulation of abnormal volumes of interstitial fluid is the
definition of oedema.
The low cardiac output
and blood pressure causes an increased sympathetic tone with constriction of
the afferent renal arterioles to the glomeruli. As a consequence, the renal
bloodflow (RBF), and the glomerular filtration rate (GFR) decrease (Fig.
10-10). Also the NaCl concentration decreases in the renal macula densa (Fig.
25-17). The renin-angiotensin-aldosterone
cascade is activated, which enhances salt-and water-retention. Angiotensin
II is a strong vasoconstrictor, which further decreases the renal bloodflow,
and aldosterone promotes the reabsorption of NaCl and water from the distal
renal system. A certain salt-water retention is beneficial in the early stages
of cardiac failure, because of improved venous return and thus improved
cardiac output according to Starlings law of the heart. However, prolonged
activation of the renin-angiotensin-aldosterone cascade and the sympathetic nervous system, damage the heart muscle further and
reduce its contractility. This is because circulating vasoconstrictors, such
as catecholamines, vasopressin and angiotensin II, imply an extra workload on
the damaged myocardium.
When the salt and water
retention results in even a small rise in the osmolarity of plasma, there is a
stimulus of osmoreceptors, located close to the neurosecretory cells in the
hypothalamus. The osmoreceptors stimulate both production and secretion of
vasopressin (antidiuretic hormone, ADH) in the neurosecretory cells. ADH eases
the renal reabsorption of water in the outer cortical collecting ducts leading
to a low urine flow (antidiuresis).
Vasopressin is also an
universal vasoconstrictor.
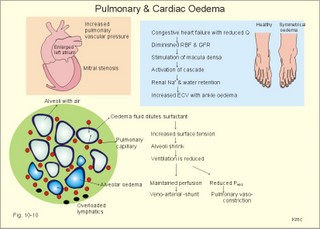
Fig. 10-10: Formation of pulmonary oedema in left ventricular failure
(mitral stenosis) and in congestive cardiac failure with ankle oedema.
Increased venous pressure
with stasis of blood dilatates the central vessels and the heart chambers. The
distended atrial wall liberates atrial natriuretic peptide (ANP), which increases Na+-excretion and dilatates peripheral vessels
(Chapter 24). This is a partial compensation of the increased preload (the water loss by high urine flow reduces venous return) and afterload (the vasodilatation reduces outflow resistance).
Venoconstriction shifts
significant quantities of blood from the peripheral to the central
circulation. Since central venous
pressure (CVP) varies inversely with TPVR,
it is possible to maintain cardiac output in resting patients with congestive
heart failure (insufficient contractile force) at the expense of increased
CVP, by reduction of TPVR (Eq.
10-2).
When cardiac output
decreases more and more during development of congestive heart failure, the
compensation fails, and both CVP and end-diastolic ventricular pressure
(preload) and volume rises further (Fig. 10-9). The superficial meck veins are
expanded, when CVP is abnormally elevated. Eventually, large volumes of plasma
water flow from the liver into the peritoneal cavity due to the elevated CVP.
Fluid accumulation in the abdomen is called ascites.
Patients with a cardiac
output much higher than normal can
develop cardiac failure. The venous return is much too high, and after some
time with an overexpansion of the heart, the cardiac pump fails to eject the
same blood volume as it receives, and an increasing blood volume is
accumulated behind the insufficient ventricle. The rise in left atrial
pressure leads to pulmonary oedema and eventually the right ventricle fails so
peripheral oedema develops.
Examples of this
condition are cardiovascular disorders with a drastic reduction of the TPVR.
The low opening pressure in the left ventricle being equal to a low
end-diastolic aortic pressure (Fig. 10-11) illustrates the low TPVR.
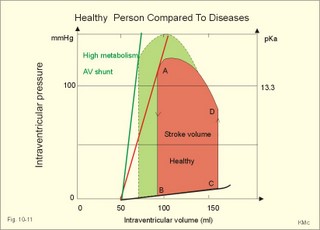
Fig.
10-11: Left ventricular pressure-volume loops from a healthy person, and from
a person with high metabolic rate (hyperthyroidism) - or a person with
arteriovenous shunts.
In hyperthyroidism (a disease with an abnormally high metabolic rate
described in Chapter 28), all the vessels in the systemic circulation
dilatate, and the venous return overloads the heart. On the other hand,
short-term administration of L-thyroxin to patients with chronic heart failure
improves cardiac and exercise performance.
Any major arteriovenous
shunt leads a large fraction of the arterial blood directly into the
veins. This greatly increases venous return and overloads the heart (Fig.
10-11).
4) Cardiogenic shock -
terminal pump failure - is such a severe reduction of cardiac output that the
peripheral tissues suffer seriously from lack of oxygen, the cells deteriorate
and within hours or days the patient die. The pulmonary capillary wedge
pressure is normal or elevated in contrast to other types of shock (blood loss
or vasodilatation).
During
insufficient pumping capacity and cardiac arrest, the cardiac pump do not get
rid of the blood volume received and a large blood volume is therefore
accumulated in the distensible venous system, pulmonary system and the
thin-walled chambers of the heart. This is why the lower part of a newly
diseased body is filled with blood in distensible vessels (livores), and the upper part of the body is pale.
I
b. Peripheral arteriosclerosis
Peripheral arteriosclerosis refers to atherosclerosis and other changes of the large and medium large
peripheral arteries. The muscular lamina media grows and becomes fibrotic
often with atheromas in small arteries. The walls of the elastic arteries
become thick of hyaline and the lumen narrows, which causes systolic
hypertension. The narrowing also leads to ischaemia, which further promotes
systolic hypertension.
The most frequent of
these disorders is chronic ischaemia of the legs, also called intermittent
claudication from its prominent symptom. Claudication is a cramp-like
pain, which occurs during exercise and subsides at rest. Occlusive
atheromatous lesions between the common iliac and the common femoral artery
lead to claudication of the thigh and calf. Lower femoral artery disease
usually causes claudication of the calf, and occlusive lesions of the
popliteal artery causes claudication of the calf or the foot.
If possible, regular
relaxed exercise should be undertaken in an attempt to develop anastomoses. In
severe ischaemia there is pain at rest. Balloon dilatation is often useful,
and amputation may become necessary.
II.
Rheumatic Heart Disease
Rheumatic fever is
caused by repeated pharyngeal infections with group A streptococcus. An
autoimmune reaction is triggered by the streptococci and the patient develops
fever, joint pains, diastolic mitral murmur caused by mitral valve
inflammation, cardiac enlargement with pericardial effusion and pericarditis
(raised ST-segment in ECG – see Chapter
11) or myocarditis (inverted
T-waves). More than 50% of those who suffer from acute
rheumatic fever with carditis develop rheumatic heart disease many years
later.
The rheumatic valvular
disease mainly affects the mitral and the aortic valves.
Mitral stenosis and regurgitation
Practically all cases of
mitral stenosis are caused by rheumatic heart disease. Severe mitral valve
stenosis is present, when the mitral valve orifice is reduced to 10-4 m2 as compared to the normal area of (5 *10-4 m2).
The left atrium dilatates and its walls hypertrophy in order to maintain
sufficient bloodflow to the left ventricle. Obviously, the pressures also
increase in the entire pulmonary vascular bed: veins, capillaries, arteries
and right ventricle.
The stenotic mitral valve
and the resistance of the pulmonary arteries determine the pressure in the
pulmonary capillary bed. Up to a certain point, pulmonary arteriolar
vasoconstriction protects the patient from pulmonary oedema.
Patients with severe
mitral stenosis have cyanotic cheeks and ears (mitral faces) caused by stasis
of the blood. Auscultation at the apex, lying on the left side just following
exercise reveals a split second
heart sound with a mitral snap (second
component) as the mitral valves open, then a mid-diastolic rumbling murmur -
like a sack of potatoes falling on a floor - caused by turbulence through the
narrowed orifice. The murmur ends in a loud first heart sound, because the
cusps are kept open until the start of the ventricular systole (Fig.
10-12).
As the left atrium grows it becomes activated later than the right, and the
P-wave is bifid (P- mitral). The large left atrium favours the development of
atrial fibrillation with thrombo-embolism and emboli to the brain, kidneys and
gastrointestinal tract.
Mitral stenosis is often
combined with regurgitation. Regurgitation is recognised by a systolic murmur
without any 1.heart sound. The 1.heart sound is caused by the closure of the
cusps, and in mitral stenosis they do not close. Usually the condition is
asymptomatic for decades following rheumatic fever. Light pulmonary oedema
presents itself as coughing and exertion dyspnoea.
Replacement of the mitral
valve is performed with artificial valves, which may work for decades with
adequate anticoagulant therapy.
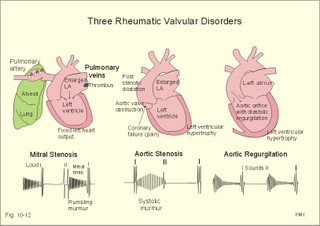
Fig. 10-12: Mitral stenosis,
aortic stenosis and aortic regurgitation.
Aortic stenosis and regurgitation
Disorders of the normal
tricuspid aortic valve are mainly caused by rheumatic fever or by
atherosclerosis. Almost half of all cases of rheumatic heart disease include
the aortic orifice, usually associated with the mitral orifice.
Symptoms and signs are
characteristic: Exercise-induced syncope and angina. This occurs when the
disease is severe and the area of the aortic orifice is reduced to 1/3 of
normal. The left ventricular pressure rises and the left ventricle
hypertrophies. The increased oxygen demand of the myocardium leads to
ischaemia with angina pectoris, arrhythmias and left ventricular failure.
Healthy persons can increase their cardiac output by a factor of 5 or more,
but this is not possible for patients with severe aortic stenosis. The
arterial blood pressure falls, the patient is pale (aortic
face), chest pains worsen, and the patient may lose consciousness. There
is a strong systolic murmur in the aortic area and over the carotid arteries.
The echocardiogram demonstrates thick aortic valve cusps and left ventricular
hypertrophy. A ventricular-aortic pressure gradient above 6.7 kPa (50 mmHg)
measured by cardiac catheterisation, is indicative of surgery. Without
surgical intervention death frequently ensues within a few years from the
occurrence of the first serious signs.
Tricuspid stenosis and regurgitation
This is an uncommon
complication, which is related to rheumatic heart disease or is congenital.
Regurgitation is more frequent than stenosis, but often the two conditions are
combined.
Isolated tricuspid stenosis dilatates the right atrium and the caval veins, and the liver swell just
like the condition with constriction of the heart. Atrial fibrillation is
frequent from the dilatated chamber.
Tricuspid regurgitation is a condition where the right ventricle delivers blood to both the pulmonary
artery and the right atrium at each systole. The right heart is dilatated,
whereas the lung vessels are not. Pulsation of the neck veins and a large
tender liver are typical signs. There is often a blowing systolic murmur over
the sternum.
Replacement of the
tricuspid valve is performed with artificial valves.
Left ventricular hypertrophy
Left ventricular
hypertrophy is an abnormal increase of the left ventricular mass caused by
increased demands of cardiac work. If due to pressure overload it is called concentric hypertrophy in which new contractile elements are lined up in parallel,
and if due to volume overload it is called eccentric hypertrophy, in which new contractile elements elongate the myocardial cell. -
This disease is described in Chapter 11.
Other
cardiovascular disorders
Thrombo-angiitis obliterans (Buergers disease). Buerger´s
disease occurs in small arteries of the limbs of young smokers –
typically males. The vessel wall is inflamed, but many lesions look like
atherosclerosis. The patient is invalid by intermittent claudication, and the
only choice of treatment is to stop smoking.
Raynaud´s disease is a
condition with cold precipitated attacks of spasms of the small arteries and
arterioles, supplying the fingers and toes. The disease is usually bilateral
and affects predominantly young girls and female smokers.
First the skin becomes
pale and white from vasoconstriction, due to slow bloodflow, and finally red
because of hyperaemia. The vasoconstriction occurs in the digital arteries,
arterioles and skin capillaries. A few minutes later the capillary smooth
muscle spasm is released due to local vasodilatators, and the capillaries are
filled with oxygen poor blood (the skin becomes blue and is still cold).
Finally, the arterial and arteriolar constriction is released and the
classical physiological reactive hyperaemia occurs, with red, warm fingers and paresthesia (numbness). Centrally
controlled vasoconstrictor tone, sensitive to cold signals, is probably
implicated in cases with symmetrical spasms. A centrally increased
vasoconstrictor tone involving the coronary bloodflow is consistent with the
fact that some of the Raynaud patients also suffer from chest pains (angina pectoris) and migraine.
Raynaud´s disease occurs
in a primary and a secondary form. Primary Raynaud´s disease is a condition
where the cause is unknown (ie essential). There is a benign familiar
occurrence of so-called dead fingers (ie, digiti mortui familiaris), and a malignant form with symmetrical gangrene
(ie, symmetric gangrene).
Secondary Raynaud´s
disease (Raynaud´s phenomenon)
occurs together with connective tissue disorders (dematomyositis,
polymyositis, systemic lupus erythematosus and systemic sclerosis). Raynaud´s
phenomenon is also a side effect to treatment with b-blockers,
in which case they must be withdrawn. The patient has to wear warm clothes in
order to protect both the shell and the core temperature. Nifedipine is sometimes beneficial.
Varicose veins have
incomplete valves. Normally, the muscular venous pump maintains the venous
bloodflow towards the heart. Patients with defective valves can develop venous
pooling or stasis and ankle oedema. This is because the contracting leg
muscles squeeze the blood in the retrograde as well as in the anterograde
direction.
Equations
Ventricular stroke work rate is the sum of the pressure-volume work and
the kinetic work:
Eq. 10-1: Stroke work rate = [(P × V) + ½ m * v2].
Both the pressure-volume work and the kinetic work are work per stroke
duration or time unit that is comparable to work-rate or effect in Watts.
The driving pressure for the systemic
circulation is the mean aortic pressure (MAP) minus CVP. The relationship to
cardiac output and total peripheral vascular resistance (TPVR) is given by:
Eq.
10-2: Cardiac output = (MAP - CVP)/TPVR.
A small fraction of TPVR is found in the venous system. The venous return
is expressed by the approximative equation:
Eq.
10-3: Venous return = (venule pressure - CVP)/ venous resistance.
Self-Assessment
Multiple Choice Questions
I. The following five
statements have True/False options:
- Smoking has no
effect on Buerger´s disease.
- When the brain
is deprived of new blood, gray out occurs 4.5 seconds following blockage
of its bloodflow.
- There is a
mid-systolic rumbling murmur at the apex in severe mitral stenosis.
- The central
venous pressure varied inversely with the total peripheral vascular
resistance.
- Organic
nitrates dilatate constricted coronary arteries, improve the bloodflow to
the subendocardial part of the myocardium especially, and dilatate
resistance and capacitance vessels.
II. The following five statements have True/False options:
- Exercise-induced
syncope and angina is characteristic of aortic stenosis and regurgitation.
- Concentric
ventricular hypertrophy is due to volume overload, whereas eccentric
hypertrophy is due to pressure overload of the ventricles.
- IDL particles
are further hydrolysed by LPL, resulting in slightly higher density of the
so-called low-density lipoprotein still maintaining its apoprotein B-100.
- Echocardiography
shows that the short ventricular radius is reduced by 15-20 mm during
systole in healthy persons at rest.
- The residual
ventricular volume is
approximately 150 ml of blood in healthy persons at rest.
Case History A
Three years following heart-lung transplantation, a patient is examined
at the hospital for the cause of frequent exertion syncope. Heart catheterisation reveals that the systolic/diastolic pressure in
the left ventricle is 26.7/0 kPa (200/0 mmHg), and in the aorta 10.7/6.7 kPa
(80/50 mmHg).
1. What
is the cause of exertion syncope?
2. What
is a likely diagnosis?
3. Argue
for the size of the left ventricular cavity and wall thickness.
Case History B
A 24-year old sporty male consults the doctor because of syncope while
playing handball.
The examination reveals a systolic murmur to the right in
the second intercostal space aortic site). The systolic murmur is audible also
over the neck. The arterial blood
pressure is 145/85 mmHg (19.3/11.3 kPa). There is a history of rheumatic fever
at the age of 11.
ECG shows a deep S-wave in V1 and a high R-wave in V6 (the sum is 5 mV), and there are asymmetrically negative T-waves in V4-V6.
1. What
is the most likely diagnosis?
2. What
is the ECG diagnosis
3. Describe
the prognosis and the therapy.
Case History C
A 55-year old female complains of headache and an arterial blood pressure
of 190/100 mm Hg (25.3/13.3 kPa) is found. Her ECG shows deep negative S-waves
in V1 and V2, and high R-waves in V5 and V6.
The sum of one S- and one R-wave is above 4 mV.
1. Calculate
the mean arterial pressure and compare the result to a normal value.
2. What
is the diagnosis?
3. Is
the patient suffering from any cardiac disease?
4. Why
is the arterial pressure amplitude (eg, systolic minus diastolic pressure)
much larger than normal?
Case History D
A girl, 17 years of age, with frequent episodes of acute tonsillitis and
articular pain during her childhood, developed a cardiac disease. The diagnosis was made as the doctor heard a
diastolic murmur over the precordial area. The main complaint of the girl was
dyspnoea at exertion. Cardiac catheterisation revealed a mean pressure in the
pulmonary artery of 58 mmHg (7.7 kPa), and in the left atrium 28 mmHg (3.7
kPa), whereas the pressure in the left ventricle was only 2 mmHg (0.27 kPa) in
early diastole. The pressure in the aorta was 105/80 mmHg (14/10.6 kPa). The
cardiac output was measured at rest with the Fick principle to be 2.5 l min-1.
Her circulating blood volume is somewhat less than her total blood volume, and
exactly 5000 ml. The blood volume of the systemic capillaries is 3% of 5000
ml.
1. What is the name of the condition with frequent episodes of
tonsillitis?
2. Calculate the pulmonary vascular resistance.
3.In what orifice is the cardiac disorder located?
4. Calculate the mean passage time in the systemic capillaries.
Case History E
A female, 33 years old, complains of attacks of severe pain in the
fingers of both hands, when she is outdoors in cold weather. She is a heavy
smoker since the age of 16. The patient also suffers from another pain
disorder. She has half-sided headache with visual disturbances at least twice
a month in the cold season. The patient explains the pain attacks in the
fingers as follows. First the skin of both hands and the fingers (not the
thumb) becomes pale and white, and they feel like dead. A few minutes later
the skin is blue, and the pain is severe. After 5-10 minutes the skin suddenly
becomes red and it is very painful.
1. What
is the diagnosis of the finger disease?
2. Are
the finger disease and the headache related?
3. Describe
the pathophysiology of the finger disease.
Try to solve the problems
before looking up the answers.
Highlights
· The
ventricular stroke work is the sum of the pressure-volume work and the kinetic
work.
· A
good index of the afterload is the peak aortic pressure during systole.
· A
ventricular-aortic pressure gradient above 6.7 kPa (50 mmHg) is indicative of
surgery in aortic stenosis.
· The
venous pump is defined as all local external forces that facilitate venous
return to the heart.
· The
skeletal muscular venous pump is also called the peripheral venous heart,
because its force must be equal to or larger than that of the heart in order
to return the blood in the upright position.
· During
inspiration, the intrathoracic pressure becomes more negative, and blood is
sucked into the large thoracic veins facilitating venous return to the heart.
· Atherosclerosis
is a process of progressive lipid accumulation (atheromatosis) and
calcification of the inner arterial walls in the abdominal aorta, lower
extremities and the arteries of the heart, brain and kidneys.
· High-density
lipoproteins (HDLs) in plasma are disk formed particles, mainly produced in
the liver for cholesterol transport. HDLs protects against the development of
atherosclerosis as they transport the cholesterol back to the liver, where the
elimination begins.
· Acute
myocardial infarction renders the heart incapable of pumping the minimal blood
volume required to transfer sufficient oxygen to the mitochondria at rest.
· Cardiogenic
shock - or terminal pump failure - is such a severe reduction of cardiac
output that the peripheral tissues suffer seriously from lack of oxygen, the
cells deteriorate and within hours or days the patient die. The pulmonary
capillary wedge pressure is normal or elevated in contrast to other types of
shock.
· Left
ventricular hypertrophy is an abnormal increase of the left ventricular mass
caused by increased demands of cardiac work. If due to pressure overload it is
called concentric hypertrophy in which new contractile elements are lined up
in parallel, and if due to volume overload it is called eccentric hypertrophy,
in which new contractile elements elongate the myocardial cell.
· Cardiac
oedema develops during chronic cardiac failure, because the kidneys retain
fluid.
· Buerger´s
disease occurs in small arteries of the lower limbs of young male smokers. The
vessel wall is inflamed, but many lesions look like atherosclerosis. The
patient is invalid by intermittent claudication, and the only choice of
treatment is to stop smoking.
· Raynaud´s
disease is a condition with cold precipitated attacks of spasms of the small
arteries and arterioles, supplying the fingers and toes. The disease is
usually bilateral and affects predominantly young girls and female smokers.
· Rheumatic
fever is caused by repeated pharyngeal infections with group A streptococcus.
The streptococci trigger an autoimmune reaction and the patient develops
fever, joint pains, diastolic mitral murmur caused by mitral valvulitis,
cardiac enlargement with pericardial effusion and pericarditis or myocarditis.
· More
than 50% of those who suffer from acute rheumatic fever with carditis develop
rheumatic heart disease many years later.
· The
rheumatic valvular disease mainly affects the mitral and the aortic valves.
· Practically
all cases of mitral stenosis are caused by rheumatic heart disease. Severe
mitral valve stenosis is present, when the mitral valve orifice is reduced to
10-4 m2 as compared to the normal area of (5 *10-4 m2).
· Disorders
of the normal tricuspid aortic valve are mainly caused by rheumatic fever or
by atherosclerosis. Almost half of all cases of rheumatic heart disease
include the aortic orifice, usually associated with the mitral orifice.
Further
Reading
Von Spiegel T, G
Wietash and A Hoeft (1998). Basics of myocardial pump function. Thorac
Cardiovasc Surgery 46, Suppl 2, 237-41.
Return
to content
Return
to chapter 10
|