Study
Objectives
-
To define adequate stimuli
for vision, hearing and balance. To define astigmatism, cataract, glaucoma,
hypermetropia, myopia, presbyopia, colour blindness, hemianopsia, scotoma,
strabismus, and visual acuity and agnosia. To define nerve deafness,
conduction deafness, presbyacusis, nystagmus, and transportation sickness.
-
To describe the anatomy of
the eye, including the retina and sensory pathways and cortical visual
organisation. To describe the inner ear including hair cells, and the
vestibular system. To describe the eye movements, receptive fields, the
colour vision, the sound transfer, the mechanical-electrical transduction in
hair cells.
-
To calculate the correction
of refractive disorders, and the hearing loss from relevant variables given.
-
To explain the function of
photoreceptors, dark adaptation, the pupillary reflex, the sensitivity to
sounds, the travelling wave and the frequency theory. To explain the diagnosis
and pathophysiology of the following disorders: astigmatism, hypermetropia,
myopia, presbyopia, colour blindness, hemianopsia, visual agnosia, strabismus,
nerve deafness, conduction deafness, presbyacusis, nystagmus, and
transportation sickness.
-
To use the concepts in
problem solving and case histories.
Principles
-
The human eye as light perceives
electromagnetic radiation at wavelengths between 400 and 725 nm.
Electromagnetic radiation ("waves") do not require a medium for
propagation. This spectrum of wavelengths is seen in a rainbow. Light
reflected from a star traverses the empty space. Electromagnetic radiation in
any narrow band within this spectrum is termed monochromatic light.
-
The camera obscura is a simple
model of the eye. The camera obscura is a device in which a small aperture
creates a reversed image on the receptive surface. The aperture can be
extended if a convex lens is mounted in front of it. The image then produced
on the receptive surface (retina) is reversed and reduced (see textbooks in
physics).
-
A mechanical wave is a wave that
propagates by disturbing the particles of a medium. Sound waves are such
mechanical or matter waves. The laws of quantum mechanics determine their
behaviour.
Definitions
is a visual field
defect reaching the periphery of the field (see glaucoma).
Astigmatism is a refractive
disorder of the eye in which the curvatures of the cornea or lens are
different along different meridians. The different meridians therefore have
different focal distances.
Cataract is an eye disease,
where the vision is blurred by an opaque lens.
Colour blindness is a group of
recessive inherited sex-linked (X chromosome) disorders. Colour blindness is
inherited from the father - with the daughter as a carrier - to her son.
Conduction deafness is caused
by impairment of the mechanical conduction of sound into the cochlea.
Far-point (F or punctum
remotum) for the eye is the fixation point in the un-accommodated eye.
Glaucoma is a term used for eye
disorders with loss of optic nerve fibres and of the visual field. Frequently,
the cause is an increased intraocular pressure (above 25 mmHg) due to reduced
fluid outflow at the irido-corneal junction.
Hypermetropia or far-sightedness is a refractive disorder with insufficient refractive power, whereby the far
point is always located behind the eye.
Hemianopsia means loss of
vision in half of the visual field of both eyes. The loss of vision refers to
the visual filed, and thus to the contralateral half of each retina.
Myopia or near-sighted is a refractive disorder, where the patient can only foveate diverging light
waves (both from the near- and the far point). The patients usually have
elongated eyeballs.
Near-point (N or punctum
proximum) of the eye is the fixation point for the maximally accommodated
eye (ie when the lens is in its most spherical configuration).
Nerve deafness is caused by
damage of the cochlea, the auditory nerve or nucleus. There is hearing loss by
both air and bone conduction.
Nystagmus is a disorder with
abnormal involuntary movements of the eyeballs.
Presbyacusis refers to the
decline with age in the capacity of hearing high tones.
Presbyopia is called old mans
sight, because the lens loses its elasticity and hence its ability to assume a
spherical shape, so the patient cannot accommodate for near vision.
Receptive field is an area of
the visual field from which light is perceived through a certain ganglion cell
in the retina.
Scotoma or localised
blindness is an island-formed visual defect caused by a lesion of the
retina in one eye, or by partial interruption of the optic nerve (see
glaucoma). The visual field defect may be absolute or relative.
Strabismus (squint) or cross-eyed is an eye disease, where the visual axes of the two eyes do not converge on
the fixation point of the object simultaneously.
Transportation sickness or kinetosis is a disorder with vertigo, nausea and vomiting due to rapid changes in
the direction of motion.
Visual accommodation is the
rise in refractive power of the lens, obtained as the lens rounds up because
of contraction of the ciliary muscle and relaxation of zonule fibres.
Visual acuity is the resolution
capacity of the eye. Cones have a high-resolution capacity and hence a high
visual acuity, because the light is focused on the fovea, where the cones are
concentrated.
Visual agnosia or mental
blindness, is lack of the ability to combine the seen object into a concept.
Essentials
This paragraph deals with 1. The
visual system, and 2. The auditory and vestibular system.
1. The Visual
System
This system detects, transmits and
interprets photic stimuli. Photic stimuli are electromagnetic waves with
wavelengths between 400 and 725 nm. This is visible light or the adequate
(effective) stimulus for the eye.
The eyes can distinguish brightness
and colour. The photoreceptors are rods and cones located in a specialised
epithelium called the retina. In each eye the retina contains about 6
million cones and 120 million rods. In the peripheral region of the retina both
rods and cones converge on bipolar cells. The bipolar cells converge on
ganglion cells giving rise to the one million nerve fibres in each optic nerve.
In addition, there are horizontal cells and amacrine cells in the retina. They
conduct impulses laterally.
Rods and cones
Rods are most sensitive in the
dark (scotopic vision). More than hundred rods converge on each ganglion
cell. There are no rods at all in the fovea.
Cones operate best in light (photopic
vision). Cones have a high-resolution capacity and hence a high
visual acuity, because the light is focused on the fovea, where the cones
are concentrated. The high resolution is also due to the small convergence of
cones to bipolar cells in the fovea (approximately a 1:1 relationship). Cones
are responsible for colour vision. Cones are surrounded by pigment, except where
the light enters.
The eye contains chamber fluid, which
is produced by filtration and secretion in the ciliary processes. The
intraocular pressure is normally 1.3-2.6 kPa (10-20 mmHg). Increased resistance
to fluid outflow at the iridocorneal junction leads to increased intraocular
pressure with loss of optic nerve fibres- or glaucoma. In this
condition, the retinal artery is compressed at the optic disc, where it enters
the eye. This causes retinal and optic nerve atrophy which eventually results in
blindness.
A diopter is the unit for the
refractive power of a lens. The diopter (D) equals the reciprocal value
of the focal length of the lens in metre (m).
Visual accommodation is the rise
in the refractive power of the lens, obtained as the lens rounds up, because
of contraction of the ciliary muscle and relaxation of the zonule fibres.
Each object we look at has a special
target point (the fixation point), from which light passes un-refracted
through the nodal point of the eye and focuses on the fovea, creating the
sharpest possible image. The nodal point in the eye is precisely the point
through which a light beam passes un-refracted. The far point (F or
punctum remotum) for the eye is the fixation point in the un-accomodated eye (Fig.
5-1). The near point (N or punctum proximum) of the eye is the
fixation point for a maximally accommodated eye (ie, when the lens is in its
most spherical configuration). The refractive power of the lens can vary between
12 and 26 D. The accommodative power of the eye is the rise in refractive
power from the un-accomodated to the maximally accommodated condition (see Eq.
5-1). A child of 10 years has 12-14 D, a 20 year old person 10 D, and a
60 year old person only 1 D in accommodative power.
The optical distance convention
defines all distances measured from a light source to the eye, to be positive.
Thus, all distances from the eye to the light source are negative. Hence, the
distance from the nodal point of the eye to a point in front of the eye
is negative. Convex refractive media bend (convergence) in-falling light behind
the media and thus have a positive diopter. Concave lenses have refractive
powers with negative Diopters, because the focal point is in front of the lens.
Convergence or near vision occurs
when the eye focuses on an object closer than 6 m from the eye. Near vision -
even with only one eye - triggers accommodation and pupillary
constriction. The ciliary muscle and the pupillary sphincter muscle are
innervated by the parasympathetic oculomotor nerve, and the two muscles contract
simultaneously for near vision.
The visual fields of both eyes are
perceived as only one continuous visual fields (the Cyclops eye effect). This is fusion or the illusion that we are looking at the world with only one
eye.
In a healthy eye, the light from an
object in the visual scenario is focused sharply on the retina by the cornea and
the lens. Both of these refract (bend) light. The cornea has a refractive power
of 43 D, and the healthy lens has a refractive power that varies between 12-26
D. Thus the total refractive power is 56-69 D. The lens allows the
eye to accommodate, so that both near and distant objects can be focused on the
retina and thus clearly seen. When we look at distant objects with normal eyes
and relaxed ciliary muscles, the object foveates automatically. However, when we
look at nearby objects, the light is initially focused behind the retina. The
lens then rounds up, by contraction of the ciliary muscles and relaxation of the
zonule fibres (i.e., accommodation), to focus the image on the fovea.
The normotropic eye has the ideal
refractive power. Parallel light from the far point (F in the upper part
of Fig. 5-1) foveates on the retina in the un-accomodated eye. Light from
the near point (N in Fig. 5-1) in the totally accommodated eye also foveates.
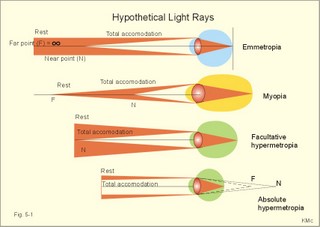
Fig. 5-1: Hypothetical light rays for
emmetropic, myopic, facultative and absolute hypermetropic eyes.
The coloured space in front of each
eye is the fraction of the three dimensional space, which can be focused on the
retina for a given visual axis (Fig. 5-1).
Eye movements
Conjugate movements are movements of
both eyes in the same direction and magnitude, so that the relation between the
visual axes is maintained. When focusing on far away objects, the parallel axes
are maintained during conjugate movements. Likewise, conjugate eye movements
maintain the convergence angles of the eye required for focusing on nearby
objects.
Saccadian or jumping
movements are rapid eye movements. Saccadian eye movement is an
instantaneous reposition of the eye that occurs when reading or when focusing on
a flash of light in the peripheral visual field. The velocity of the movement is
up to 500o per s. The latency period is 250 ms, and the contraction
time is 50 ms. The compensatory eye movement involving the vestibular system,
occurs when the head rotates. This is also an example of Saccadian eye movement.
In contrast, pursuit movements are
smooth eye movements that allow the eye to track a moving object. They have a
velocity of up to 30o per s.
These two movements work together in optokinetic
nystagmus. This is a shift between smooth pursuit movements and correcting
jumps. The direction of nystagmus is by convention indicated by the rapid
correcting phase.
Even during foveation of an object
the eyes are not totally still. The eyes are continuously performing miniature
eye movements, which occur at a rate of 3 microsaccades per s, with mean
amplitude of 0.1o.
Photoreception
The number of photoreceptors in a
human eye is estimated to be 110-130 million rods and 5-7 million cones.
Each photoreceptor cell includes an outer and an inner segment, which are united by a thin cilium. The
outer segments are directed towards the pigment epithelium of the peripheral
retina, and contain stacks of disks that are rich in photo-pigment
molecules. The inner segments contain the cell nucleus and numerous
mitochondria. The rods are predominant outside the fovea, and they
contain much more pigment (108 rhodopsin or molecules per rod) than
do cones. Rods are so sensitive that a single photon can trigger a rod response.
Rods are therefore well suited for night vision. Rhodopsin or visual purple has two absorption maxims: 350 and 500 nm. The spectral extinction curve for
rods corresponds to that of rhodopsin, suggesting that rhodopsin is the
chemopigment in rods. Rhodopsin consists of a glycoprotein (opsin) and a
chromophore group (11-cis-retinal). Retinal is the aldehyde of vitamin A1 (retinol).
The fovea only contains cones.
Cones function in the daytime with maximal visual acuity and colour vision. The
human eye possesses three types of cones, each with a specific pigment
related to the three basic colours: red (erythrolab), green (chlorolab) and blue
(cyanolab). The cones in the fovea do not contain cyanolab.
When the human eye is fully adapted
to darkness, its rods have open Na+-channels, and the resulting
influx of Na+ maintains depolarised rods with a resting membrane
potential of -40 mV. The rod cell synapses with bipolar and horizontal
cells, and releases glutamate as long as the dark depolarisation is
maintained. Na+ is continuously removed from the rod by the Na+-K+ pump.
Inside the rod a special
amplification takes place. Light absorption by a single rhodopsin molecule activates
thousands of G-protein molecules (transducin), which then activate large
quantities of cGMP phosphodiesterase in the discs. Each of these enzyme
molecules catalyses the hydrolysis of cGMP to 5’-GMP at a rate of thousands
per second. The reduction in [cGMP] closes the Na+-channels, and
hyperpolarises the cell. The amplification mechanism is probably why the eye is
capable of detecting a single photon.
A similar cascade of reactions takes
place in cones, when they are stimulated. Cones are so small that the
hyperpolarization occurs rapidly.
Each ganglion cell has a receptive
field in the retina that is comprised of a number of photoreceptors.
The fraction of a receptive field
belonging to each photoreceptor is added to neighbour areas in order to obtain
the receptive area of a bipolar or a horizontal cell. An on-bipolar cell is
depolarised by white light, whereas an off-bipolar cell is hyperpolarised.
Signals are transmitted from the photoreceptors to the ganglion cells as a
graded response. These small receptive areas are summated to form a circular
receptive field for each ganglion cell (Fig. 5-2). Ganglion cells can generate
action potentials and transmit signals to the brain.
-
One type of ganglion cell has
a centrally located excitatory area, surrounded by an inhibitory annular
area (Fig. 5-2). Together these form an on-centre off-surround receptive
field. Here, an on-response is triggered in the bipolar cell that is
connected to the on-ganglion cell.
-
Another type of ganglion cell
has a centrally located inhibitory area (inhibited by light), surrounded by
an excitatory annular area (Fig. 5-2). These form an off-centre
on-surrounding receptive field.
-
A third type of ganglion cell
is connected to both on- and off-bipolar cells, so its centre is both
stimulated and inhibited by white light.
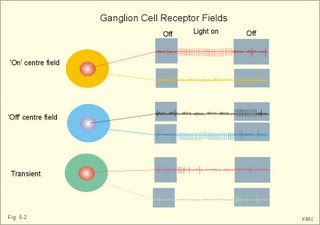
Fig. 5-2: Ganglion cell receptor
fields in the retina.
The ganglion cells can also produce transient or sustained reactions. These reactions are due to adaptation to
light (decreased sensitivity with exposure) and lack of adaptation, respectively
(Fig. 5-2).
Ganglion cells in the fovea are
connected to few or only one cone. Some ganglion cells are excited by blue light
and inhibited by its opponent colour yellow. Other cells are excited by green
and inhibited by the opponent colour red. This mechanism is the so-called colour
contrast analysis of the retinal ganglion cells. Colour opponent neurons are
found not only in the ganglion cells but also in the lateral geniculate nuclei.
Retinal signals pass through the main
visual pathway: the optic nerve, the lateral geniculate nucleus, the optic
radiations (the geniculostriate tracts), the primary visual cortex, the
pretectal area, the Edinger-Westphal nucleus, the oculomotor nerve and the
ciliary muscle.
Each point of the retina has a
corresponding location in the dorsal lateral geniculate nucleus and in the
visual, striate cortex (area 17). The nerve fibres in the optic nerve run so the
upper quadrants of the retina are represented in the upper half of the nerve,
and the lower quadrants in the lower half.
Such a retinotopic map is
present in the lateral geniculate nucleus and maintained throughout the
visual pathways and in the visual cortex. The receptive field in the retina is
maintained all the way to the cortex. This is the basis of fusion. The
consequence is that the right striate cortex receives information about
objects located in the left side of the visual field, and the striate cortex in
the left hemisphere receives information about the right side of the field of
vision. In general, each hemisphere of the brain is connected to sensory and
motor activity of the opposite side.
The lateral geniculate nucleus has
three different pairs of neuronal layers (1-2, 3-4, 5-6). Ganglion cells from
the ipsilateral (same side) eye projects to layers 2, 4 and 6, whereas ganglion
cells from the contralateral eye projects to layers 1, 3, 5. The lateral
geniculate nucleus is involved in integration and registration of pictures
formed in corresponding areas of the retinal surfaces. Some neurons react to
white light (with circular receptor fields), while other neurons react to
opponent colours. When we jump from one highlight to another in the visual
field, each jump is called a saccade. Selection of visual stimuli may be located
in the lateral geniculate neurons (possibly performing gate control).
Most of the neurons in the geniculate
nucleus projects to the striate cortex by way of the optic radiations (geniculostriate
tract). Neurons in a certain column of the lateral geniculate nucleus project to
precisely the same part of the striate cortex (area 17). The lateral geniculate
nucleus also receives information from the cortex (in particular the visual
cortex) that is essential for selection of signals of particular interest.
The striate cortex (area striata,
area 17) is located around the calcarine fissure on the medial side of each
occipital lobe. The optic radiation ends mainly in synaptic contact with simple
cells in layer 4 of the striate cortex. Simple cells have on- and off-fields.
Complex cells receive inputs from several simple cells, and hypercomplex cells
receive inputs from several complex cells.
Axons from one eye terminate in
millions of functional units called ocular dominance columns consisting of about
103 neurons. Cortical neurons are arranged in orientation columns
showing orientation selectivity for lines edges or bars. Other cortical neurons
are arranged in direction columns showing direction selectivity. Colour blobs
are interspersed among the other columns (see later).
A large area at the occipital pole
represents the macula, and the upper and lower half of the visual field is
represented below and above the calcarine fissure. The upper layers of the
superior colliculus perform visual processing. The deep layers produce eye
movements.
The cortical area V4 contains colour-sensitive
neurons, and the visual association areas 18 & 19 (Fig.
4-4) contain many
cells with complex functions.
The absolute sensitivity depends upon
the adaptive condition of the retina, the pupillary diameter, and the source of
light (spectral composition, exposure time, and light source dimensions). The
threshold for the completely dark-adapted eye is (7× 10-11) Watts/m2.
The
Trichromatic Theory
Light adaptation is a decrease in
visual sensitivity during constant stimulation. This occurs rapidly because the
rhodopsin bleaches readily. Hence, in daylight (photopic cone vision) we are
dependent on cones for vision. Night vision (scotopic rod vision) is extremely
sensitive to light, because of dark adaptation. It takes at least 20 min in dark
surroundings before the rods become fully adapted. In a dark movie theatre, we
have scotopic vision with low visual acuity and colour blindness. As soon as the
film is projected we experience partial light adaptation, so that the photopic
cone vision is resumed.
The trichromacy theory postulates
that an appropriate mixture of the three basic colours can produce any colour:
red, green and blue. The three types of cone pigments have different opsins, and
opsins that differ from that in rhodopsin. Groups of cortical neurons called
cortical colour blobs respond specifically to colour signals, and also
receive signals from adjacent columns of the visual cortex. Cortical colour
blobs are probably the primary stations for perception of colour, and they
are found both in the primary and the secondary visual cortex areas. Perception
of spectral opponent colour pairs is located in discrete colour blobs of the
visual cortex.
The three cone pigments are
Erythrolab for red (maximal sensitivity at 555 nm), chlorolab for green (525
nm), and cyanolab for blue (450 nm). The absorption spectra of the photopigments
overlap considerably. The three cone types are uniformly distributed in the
retina, except in the fovea. Fovea has no cyanolab cones and no rods.
This gives the fovea partial physiologic scotoma (ie, no blue vision and no
scotopic dark vision). The real physiologic scotoma is the dark spot corresponding to the optic papilla. Inhibition of neighbour ganglion
cells from on centre field ganglion cells is called lateral inhibition; it
occurs also in the lateral geniculate nucleus or in the visual cortex. Lateral
inhibition provides simultaneous contrasts and enhancement. Each colour-contrast
neuron is excited by one colour and inhibited by the opponent colour. Opponent
colours are red-green, yellow-blue, and green-purple.
Contrast analysis begins already in
the retina and is elaborated centrally in the lateral geniculate nucleus, the
thalamus and the visual cortex. If there is a multilevel neural system for the
analysis of colour mixing, we also need to assume the existence of a neural
system for colour brightness, depending upon the intensity of the light. Healthy
people are trichromats, because they have all three cone pigments.
Spatial resolution or minimum
separabile is the capacity of the eye to see two stimulated retinal areas as
separated. In healthy young humans the spatial resolution is about 1/60 degree,
depending upon luminosity, exposure time, patterns and opponent colours in the
visual scenario. The most important factor limiting this capacity is the
cerebral integration.
Temporal, visual resolution is the
capacity of the eye to see consecutive light stimuli as separate. Intensity is
directly related to duration of perception of light. Contrast further decreases
temporal resolution, a flash of light in the dark is perceived for longer than
in bright surroundings. Temporal resolution is also determined by the wavelength
of light. The eye is maximally sensitive at the absorption maxims of the three
cone pigments and rhodopsin.
The positive after-picture is a
visual impression lasting longer than the stimulus. It is visible on a dark
background following exposure of the eye to intense light. The negative
after-picture follows the positive afterpicture as a dark shadow or as the
opponent colour. The negative after-picture is due to adaptation of the area in
the retina related to the picture.
A flickering source of light
liberates successive flashes so rapidly that they fuse, and appear to be
continuous. In the darkness of a movie theatre we do not sense the flickering
frequency of 24-48 frames each s, or those of a television screen with 50-60
frames per s. With increasing intensity of illumination the critical fusion
frequency increases abruptly. This is why young persons can look directly into a
neon light and see its flickering character even with 60-100 flashes each s.
Accordingly, the cones of the healthy human eye have a critical fusion frequency
around 60-100 flashes per s with optimal illumination. The photopic cones are
much more sensitive to rapid alterations of light intensity than the rods.
Movements in the visual scenery are
depicted as opposite movements on the retina. Convergent inputs from the eyes
result in depth perception (ie, stereopsis or stereoscopic vision). Stereopsis
depends upon the medial, longitudinal fasciculus and the corpus callosum. These
structures co-ordinate the movements of the two eyes. The two eyes are 7-8 cm
apart, which causes slight disparities between their retinal images. Disparate
receptive fields and thus excitation of specific cells in the secondary visual
cortex probably exhibit the perception of depth.
Distance evaluation requires high
visual acuity and experience with objects of known size.
Essential for the development of the
baby’s brain is human milk proteins and long chain fatty acids in the mother
milk. Protein deficiency from birth reduces formation of brain neurons and thus
limits brain development including the development of visual capacity. Many
vitamins and key proteins have hormonal and transmitter function in the brain,
and lack of such substances in the critical growth period just after birth,
results in irreversible damage. The action of endogenous nerve growth factor is
necessary for the normal functional and anatomical development of the visual
system. In the critical period of visual development, which is the first two
years of life, the child must be exposed to a multitude of visual stimuli. This
is necessary for the development of neurons and key substances that can record
future visual stimuli. The ability to fuse the two optic fields is a process
that has to be practised. This fact is an important basis for the treatment of
cross-eyedness (strabismus).
Cross-eyedness or squint (strabismus)
is an eye disease, where the visual axes of the two eyes do not converge on the
fixation point of the object simultaneously. Thus the retinal images do not fuse
on corresponding areas on the two retinas. Since the fixation line only foveate
in one eye, the patient can learn to suppress the other picture in the brain.
Hereby, double vision is avoided at the expense of visual acuity.
2. The
Auditory And The Vestibular System
The two systems share the labyrinth,
and transmit signals to the brain through the 8th cranial nerve. The
two systems record fluid movements and use the so-called hair cells as
mechanical transducers.
Sounds are sense impressions that
consist of complex mixtures of compression and decompression waves that can be
broken down to pure tones by Fourier analysis. Pure tones are sinusoidal waves
of a specific frequency (cycles per s or Herz = Hz) and amplitude.
Sinusoidal waves can change phases.
The normal human ear is sensitive to pure tones with frequencies between 10 and
30 000 Hz, in a young person.
As people age, their capacity to hear
high tones declines. This condition is termed presbyacusis.
Sound propagates at 343 m/s in air at
20oC, although each single air molecule only moves a few mm
in the direction of propagation. The unit of sound pressure (p) is Pascal (Pa).
According to international convention the sound pressure level (SPL) is
expressed in decibel (dB) - see Eq. 5-2.
Any rise in the SPL of 10 dB implies
a rise in sound pressure by a factor of 3, since the log of 3 is 0.5: 10 dB = 20
log 3 (Eq. 5-2).
Speech has an intensity of 60-65 dB,
and sounds that exceed 100 dB can damage the ear. A constant sound stimulation
only results in minor adaptation. The human ear has the largest sensitivity
around 1000-4000 Hz, the range for normal speech.
The sound pressure waves in air are
converted into sound pressure waves in the fluid column within the cochlea. The
pressure wave in the air is transmitted via the tympanic membrane and the
ossicles (malleus, incus and stapes), to the fluid of the cochlea. The foot
plates of the stapes inserts in the oval window, and separates the middle ears
from the fluid of the cochlea. The ratio of the effective surface area of the
tympanic membrane to that of the oval window is 14:1, and the pressure is
increased further by the differing lengths of the lever arms in the chain of
ossicles. By this area-pressure amplification, hearing is improved by more than
25 dB.
When the external ear is filled with
water during diving, hearing is seriously reduced.
Two muscles are found in the middle
ear. They dampen movements of the ossicular chain when the ear is exposed to
extremely high pitch sounds that can be anticipated. These muscles are the
tensor tympani muscle supplied by the trigeminal nerve, and the stapedius muscle
supplied by the facial nerve. Exposure to sounds above 90 dB elicits reflex
contractions.
The cochlea is composed of three tube
systems coiled together to form a pyramid: scala vestibuli, scala media and
scala tympani (Fig. 5-3). The part of cochlea beneath the oval window is called
scala vestibuli, and it is filled with a fluid column termed perilymph.
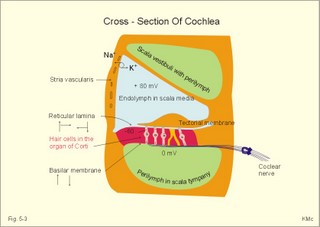
Fig. 5-3: A cross section through
one of the turns of the cochlea.
The perilymph conducts the pressure
wave to the basilar membrane, which is displaced within the endolymph together
with the whole organ of Corti, which contains the hair cells.
Each hair cell has 40-100 hairs (stereocilia).
The hairs have different heights, and when the pressure wave displaces the hairs
towards the tallest hair, the hair cells are depolarised. When the basilar
membrane moves upward towards the scala media, the reticular lamina shifts
upward and inward (Fig. 5-3), causing the hair cells to depolarise. Downward
movement of the basilar membrane towards the scala tympani moves the reticular
lamina downward and outward (Fig. 5-3). This movement hyperpolarises the hair
cell membrane.
The endolymph in the scala media has
a potential difference of +80 mV with the perilymph as reference. The inside of
the hair cell is -60 mV compared to the perilymph; this is a resting membrane
potential about the same size as in most neurons. Thus the total potential
difference between the inside of the hair cell and the endolymph in the scala
media is -140 mV. This resting membrane potential is maintained by Na+-K+-pumps
in the Stria vascularis (Fig. 5-3).
Bending of the hair change the
conductance of K+-ions through the apical hairy membrane, and this is
how the resting membrane potential is changed. A current flow is produced
through the hair cell from apex to base, which is resting on the basilar
membrane (Fig. 5-3). This current flow or receptor potential can be recorded
extracellularly with microelectrodes as the cochlear microphone potential (ie,
the sum of receptor potentials from many hair cells). This potential has the
same frequency as the acoustic stimulus, and the potential is analogous to the
output voltage of a microphone. The cochlear microphone potential follows the
sound stimulus without latency, without measurable threshold, and without
fatigue in contrast to neuronal action potentials.
Stimulated the hair cells release
neurotransmitters (glutamate, aspartate) that excite the cochlear nerve fibres.
Thus, the propagating action potentials are generated in the cochlear nerve
fibres.
A high frequency tone produces
travelling waves along the basilar membrane. High tones travel only a short
distance from the stapes along the basilar membrane to their resonant point,
where the displacement amplitude of the basilar membrane is maximal (Fig. 5-4).
Low frequency tones travel all the way to the apex of the cochlea (Fig. 5-4).
The higher the tone frequency, the more basal located in the cochlea is the
resonant point and its potential.
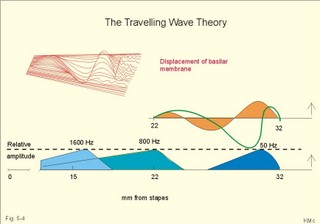
Fig. 5-4: Displacement of the basilar
membrane illustrates the travelling wave theory (von Bekesy).
The existence of such a maximum of
the travelling wave is termed frequency dispersion. Since different
frequencies excite differently located hair cells the argument is called the
place analysis theory. The brain also utilises the temporal structure of the
sound stimulus. This is the so-called periodicity analysis.
The receptor potentials generate
action potentials in the cochlear nerve (8th cranial nerve) that
travel to the cochlear nuclei. Secondary neurons transfer the signals from here
to the superior olivary nuclei that co-ordinates the two ears, or directly to
the inferior colliculus through the lateral lemniscus (representing both ears).
Axons from the inferior colliculus ascend to the medial geniculate nucleus of
the thalamus. Axons from this thalamic nucleus form the auditory radiation,
which terminates in the auditory cortex in the superior temporal gyrus (areas 41
and 42 in Fig. 4-4). High frequencies are projected to the rostral auditory
cortex, and low tones to the caudal section.
The duration of a sound stimulus is
encoded in the duration of the neural signal, and its intensity by the level of
neural activity.
Projections from the auditory cortex
also descend to the medial geniculate nucleus and the inferior colliculus. The
oligocochlear bundle controls several sound impressions. Efferent stimulation
through these pathways inhibits the sensitivity of these nuclei for sounds,
while increasing their tone selectivity. This phenomenon, and a high degree of
motivation, explains how a mother can hear her baby cry in spite of noise, and
also how we can hear an individual in a crowd (the cocktail party effect).
Localisation of a sound source
depends upon the difference in time between the arrival of a low frequency sound
signal to the left and right ears (time delay). The sources of low frequency
sounds (below 2000 Hz) are localised by this time delay. The source of high
frequency sounds is localised by the difference in sound amplitude arriving at
each ear caused by the dampening of the sound intensity.
Sounds in the region of 2000 Hz
cannot be detected by either mechanism. On average, the distance between the two
organ’s of Corti is about 0.16 m. Then, a sinusoidal wave or pure tone with
exactly the same wavelength coming from one side of the head, is in phase when
they reach the ears. This wavelength corresponds to the frequency of 2144 Hz
(343 m/s divided by 0.16 m). In this instance the subject will be unable to
determine the source of the sound.
The vestibular system detects if the
body is in balance. The sensory unit of the auditory-vestibular system is the
membranous labyrinth, located in the petrous portion of the bony labyrinth. The
membranous labyrinth contains endolymph and is surrounded by perilymph; it is
composed of the auditory cochlear duct or scala media, and the balance
regulating the Vestibular system. The vestibular system consists of three
semicircular ducts and two otolith chambers (the utricle and the saccule). Each
semicircular duct has a swelling termed an ampulla (Fig. 5-5).
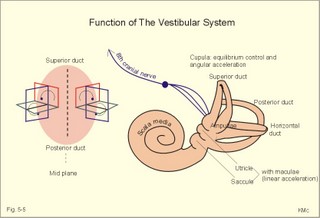
Fig. 5-5: The spatial orientation of
the three semicircular ducts in the upright person (left). The horizontal duct
is not drawn. The membranous labyrinth is shown to the right.
The semicircular ducts consist of a
horizontal duct, a superior and a posterior duct at right angles to each other,
so that they cover all three planes in space. The semicircular ducts all
communicate with the utricle. The utricle joins the saccule, which receives new
endolymph from the cochlear duct.
The sensory organ of each utricle and
saccule is called a macula. The sensory organ of each semicircular ampulla is
the crista ampularis.
Each macula contains thousands of
hair cells. Vestibular hair cells each have many stereocilia (hairs) on their
apical surface just as cochlear hair cells do; however, they also have a large
stereocilium called kinocilium. The hairs are imbedded in a gelatinous
substance, the otolithic membrane that also contains earstones or otoliths.
These otoliths increase the specific gravity of the otolithic membrane to twice
that of the endolymph. Thus their hair cells are sensitive to linear
acceleration such as gravity and to static equilibrium control, but not to
angular accelerations of the head. The macula of the utricle is located in the
horizontal plane, and the macula of the saccule in the vertical plane.
Each crista ampularis consists of
many hair cells. Here the hairs are imbedded in a large gelatinous substance
termed a cupula. The cupula occludes the lumen of the ampulla completely, and
its material has the same specific gravity as the endolymph. The cupula is
concerned with equilibrium control during motion and with angular acceleration
(rotation of the head), but is unaffected by linear acceleration.
When the stereocilia are bent toward
the kinocilium, the conductance of the apical cell membrane increases for
positive ions, and the hair cell becomes depolarised. Bending the stereocilia in
the opposite direction hyperpolarizes the cell. The depolarised hair cell
releases glutamate or aspartate and increases the discharge rate of the nerve
fibre with which it synapses.
The utricles and saccules are
sensitive to linear accelerations. When we suddenly thrust our body forward, the
otolithic membranes fall backwards on the cilia of the hair cells until the
thrust stops. Then, the otolithic membranes fall forwards. The signals to the
brain make us feel as if we were falling backwards. Therefore, we lean forward
until the otolithic membranes are in balance.
Pathophysiology
This paragraph deals with 1. Refractive disorders, 2. Colour blindness, 3. Visual field defects, 4. Mental
blindness, 5. Deafness (hypacusis), 6. Nystagmus, and 7. Kinetosis.
1. Refractive
Disorders
(myopia, hypermetropia, astigmatism,
presbyopia, and cataract).
Near-sighted (myopic)
patients usually have elongated eyeballs. More rarely, myopia can be
caused by too high refractive power in the lens system. Myopic persons can only
foveate diverging light waves - both from F and N (Fig.
5-1). The images of
distant objects are focused in front of the retina, and the image is blurred on
the retina. Both F and N are located in front of the eye. Concave lenses (-D)
accomplish correction. The weakest concave lens compatible with optimal visual
acuity is the best correction, as the accommodation is eliminated.
Hypermetropic or far-sighted
persons usually have shortened eyeballs, and F is always behind the eye.
In rare cases hypermetropia can also be caused by insufficient refractive power
in the un-accomodated eye. The absolute hypermetropic eye can only focus
images of distant objects behind the retina (Fig. 5-1). The facultative
hypermetropic eye can focus converging light beams on the retina without
accommodation (rest in Fig. 5-1). This patient can read the Snellen letters
without problems; they also foveate diverging light beams by accommodation, but
then the patient gets eyestrain due to fatigued ciliary muscles. Convex lenses
(+D) correct hypermetropia. The strongest convex lens compatible with optimal
visual acuity is the best correction, as the accommodation is eliminated.
Astigmatism is a
refractive disorder of the eye, in which the curvatures of the cornea or lens
are different along different meridians. The different meridians therefore
have different focal distances. Therefore, astigmatism can be corrected with cylinder
lenses that correct the curvature differences.
Presbyopia is called old man’s
sight. The far point remains where it is, so the un-accomodated refraction
is unaltered. The ability to accommodate is changed, so that N approaches F.
With age, the lenses of most people
loses its elasticity, and hence their ability to assume spherical shape. The
lens is the organ in our body with the highest protein concentration.
Alterations of lens proteins probably cause progressively increasing
stiffness of the lens. The accommodative power decreases from 14 D in a
child to less than 2 D at the age of 50. The patient’s eye becomes incapable
of accommodation for near vision and reading. Convex lenses correct presbyopia.
Cataract is an eye disease, where
the vision is blurred by an opaque lens. Precipitation of lens proteins
can occur in several ways. It is often due to oxidative processes. The lens
needs oxygen, but strong sun light or radiation can oxidise lens proteins
in unprotected eyes. The oxidation is enhanced by hyperbaric oxygen therapy and
by high blood [glucose] in diabetics. Oxidants in the food may be the cause in
some patients. Antioxidants, such as vitamin A and D, seem to protect against
the loss of transparency in long-term studies. Today, excellent surgical
techniques are used to eliminate the opaque lens and re-establish normal
refraction.
2. Colour
blindness
The three colour genes are located on
an X chromosome. Females have two X-chromosomes, and colour blindness is
rare among females. Colour blindness is inherited from the father - via
the daughter - to her son. The trait is recessive and sex linked. The
total incidence is about 8% of the male and 0.5% of the female population. Monochromats lack all three or two cone pigments, an extremely rare
disorder. Dichromats lack one of the three cone pigments. Proteus is the
first or red component, so protanopic people are blind for the red part
of the spectrum. They cannot separate red and yellow signals in traffic. Deuteranopic
patients are blind for the second or green colour, and tritanopics are blind for blue - the third basic colour. Abnormal trichromats have a
reduced amount of one cone pigment: Protanomalous trichromats lack
erythrolab, deuteranomalous (the most frequent type) lack chlorolab, and tritanomalous lack cyanolab.
3. Visual
field defects
Visual field defects are caused by
interruptions of the visual pathways. Hemianopsia means loss of vision in
half of the visual field of both eyes. The loss of vision refers to the visual
field, and thus to the contralateral half of each retina (shown with two colours
in Fig. 5-6).
Homonymous hemianopsia means that
the same side of the visual field for each eye is defective. Corresponding
halves of each retina has lost vision (black in the illustration). Homonymous
hemianopsia occurs from lesions of the entire optic tract, the lateral
geniculate body, the optic radiation, or the entire visual cortex of the
contralateral hemisphere (Fig. 5-6). A lesion of the striate cortex often spares
the large macular area at the occipital pole. This results in a disorder termed homonymous
hemianopsia with macular sparing (Fig. 5-6). Partial lesions may cause quadrant-anopsia.
Heteronymous hemianopsia can be
bitemporal or binasal (Fig. 5-6). Bitemporal hemianopsia results from damage of
the optic nerve fibres as they cross the optic chiasm (Fig. 5-6).
An expansively growing pituitary
tumour, perhaps related to acromegaly, can damage crossing fibres, originating
from ganglion cells in the nasal halves of each retina. Expansion of the tissues
surrounding both carotid arteries is a rarity, which can damage nerve fibres
from the temporal halves of each retina, and cause binasal hemianopsia (blind parts of the visual fields shown in Fig. 5-6 are marked with red - or with black)..
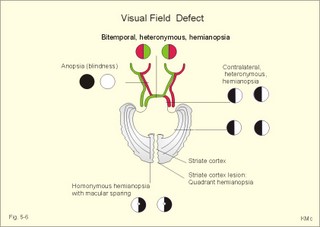
Fig. 5-6: Visual field defects.
Lesions are shown with bars.
As long as the patient sees with both
eyes, he may not experience any visual defect caused by damage to
non-corresponding areas of the retina.
Localised blindness or scotoma is caused by a lesion of the retina in one eye, or by partial interruption of
the optic nerve. Interruption of the entire optic nerve results in complete
blindness or anopsia (Fig. 5-6).
Ophtalmoscopy is an
important diagnostic tool able to establish both eye disorders and systemic
diseases.
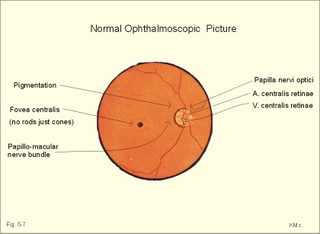
Fig. 5-7: The eye background (fundus)
of the right eye in a healthy person. – A typical hypertensive eye background
is shown in Fig. 9-6.
The normal ophtalmoscopic picture of
the fundus is seen in Fig. 5-7. The papilla is clearly visible with the central
artery and vein, and the cone-filled fovea is located to the left. The papillo-macular
nerve bundle connects to the cones of the macula, but this bundle is invisible.
This person has a small pigmented area along the lateral side of the papilla
(Fig. 5-7).
4. Mental
blindness
Bilateral temporal lobe lesions can
lead to the Klüver-Bucy syndrome. In this condition, the temporal cortex,
hippocampus and the amygdaloid body are damaged. The Klüver-Bucy syndrome
includes mental blindness (visual agnosia). Mental blindness is the
inability to recognise objects seen. Besides mental blindness, the syndrome
consists of loss of short-term memory, and hypersexual behaviour incompatible
with normal social adaptation. This hypersexual behaviour is related to the
visual agnosia.
Damage to visual areas of the
temporal cortex alone causes isolated visual agnosia. Visual agnosia or
mental blindness, is lack of the ability to combine the seen object into a
concept. This visual agnosia can be colour-agnosia (acromat-agnosia) or face-agnosia
(prosop-agnosia).
5. Deafness
(hypacusis)
Nerve deafness is caused by
impairment of the cochlea, the auditory nerve or the nucleus. Chloramphenicol,
kinin and streptomycin can damage the cochlea. These drugs can cause hearing
loss or deafness for all sound frequencies. Deafness to specific
frequencies is caused by localised damage of the basilar membrane. This is
typical for rock and beat musicians, soldiers, and airline pilots. The nerve
deaf patient has a hearing loss when tested both by air conduction through the middle ear, and bone conduction through surrounding bone
structures. A certain type of nerve deafness for high tones develops among older
persons (presbyacusis).
Conduction deafness is caused by
impairment of the mechanical conduction of sound into the cochlea. A hereditary
disease called otosclerosis is due to fixation of the faceplate of the stapes to
the oval window. Otosclerosis, blockade of the external ear with ear wax, otitis
media, damage of the tympanic membrane, and of the ossicles all cause conduction
damage to hearing. Persons with conduction damage have normal bone conduction.
6. Nystagmus
Nystagmus is a disorder with abnormal
involuntary movements of the eyeballs. Opto-kinetic nystagmus occurs when
travelling in a train or a car. The eyes remain fixed on an object long enough
in order to gain a clear image. The semicircular ducts cause the eyes to rotate
in the direction opposite to the direction of travel. Optokinetic nystagmus
involves the vestibular nuclei, the medial longitudinal fasciculus, and
the oculomotor nuclei.
Post-rotatory nystagmus is
observed in a person sitting in a rotating chair. This is the physiologic
adequate stimulus for nystagmus.
Caloric nystagmus refers to the
horizontal reflex movement of the eye when the external ear is flushed with hot
or cold water. The fast phase of the nystagmus is directed away from the ear
flushed with cold water, and towards the ear flushed with hot water. The caloric
nystagmus test is preferable to the post-rotatory test for testing the nystagmus
reflexes, because it examines one ear at a time, and is more convenient.
7. Kinetosis
or transportation sickness
Many types of transportation, which
subjects passengers to rapid changes in the direction of motion, elicit kinetosis.
Kinetosis is a disorder with vertigo, nausea and vomiting. The disorder is
triggered from the vestibular system, provided that the cerebellar
function (the flocculo-nodular lobe) is intact. The flocculo-nodular lobes are
linked to the equilibrium control of the semicircular system. Persons with
destroyed semicircular canals or with destroyed flocculo-nodular lobes can be
completely protected from kinetosis at the expense of lost equilibrium during
motion.
Equations
The accommodative power of the
eye is the rise in refractive power from un-accomodated to the maximally
accommodated condition:
Eq.
5-1: Accommodative power
= 1/F - 1/N.
F and N are the far- and the near
point, respectively.
A child of 10 years has the high
accommodative power of 12-14 D, a 20-year-old person 10 D, and a 60-year-old
person 1 D.
According to international
convention the sound pressure level (SPL) is expressed in decibel
(dB):
Eq.
5-2: Sound pressure level
(dB) = 20 log p/po.
The actual pressure is p, and the
threshold for sound pressure is po . The threshold for sound pressure
is 20 mPa in air (po) at 1000 Hz in
a sound tight chamber for a healthy person. This pressure corresponds to a sound
effect of 10-12 Watts/m2.
Self-Assessment
Multiple Choice Questions
I. The following five statements have
True/False options:
A. The fovea has no cyanolab
cones and no rods.
B. Nystagmus is a disorder with
abnormal voluntary movements of the eyeballs.
C. The cornea has a refractive
power of 43 D, and the healthy lens has a refractive power which varies
between 12-26 D. Thus the total refractive power is 56-69 D.
D. Light absorption by a single
rhodopsin molecule activates thousands of G-protein molecules (transducin),
which then activate large quantities of cGMP phosphodiesterase in the discs.
Each of these enzyme molecules catalyses the hydrolysis of cGMP to 5’-GMP at
a rate of thousands per second.
Parallel light from the far
point foveates on the retina in the fully accommodated eye.
II. The following five statements
have True/False options:
-
Nerve deafness is caused by damage
of the cochlea, the auditory nerve or nucleus. There is hearing loss by air
conduction only.
-
Kinetosis is a disorder with
vertigo, nausea and vomiting due to rapid changes in the direction of motion.
-
Drugs, such as chloramphenicol,
kinin and streptomycin, can cause hearing loss for all sound frequencies.
-
The organ of Corti contains hair
cells, each with 40-100 stereocilia. When the pressure wave displaces the
hairs towards the tallest stereocilia, the hair cell is depolarised.
-
Low tones travel only a short
distance from the stapes along the basilar membrane to their resonant point,
where the displacement amplitude is maximal.
5. Case
History A
A 30-year old female complains of
eyestrain and frontal headache during reading - sometimes followed by nausea and
vomiting.
The patient is placed 6 m (20 feet)
from the Snellen test chart. She is able to read line 6, which is the letter
size read by a normotropic eye. Now thin convex lenses are placed in front of
her eyes, but she can still read line 6. The diopter of the strongest convex
lens with which she can still read line 6 is +4 D for both eyes. These
converging light rays must be directed against the far-point (F). F must be
located behind the eyes at a distance of ¼ m. Examination with concave lenses
reveals the strongest concave lens by which she can read line 6 to be -3 D or N
= -1/3 m (in front of each eye).
-
1. What is the refractive anomaly
of the patient?
-
2. A normotropic person of 30
years has an accommodative power of 7 D. Compare the accommodative power of
the female patient to that of the normal person?
-
3. Is it possible for the patient
to read a fine text 0.2-m in front of her?
-
4. The saggital diameter of the
patient’s eyes is typical. Describe its characteristics.
5. Case
History B
A patient with a hearing loss of 26
dB is working in a power station, where the daily sound intensity is 100 dB and
the air temperature is 20oC.
1. Calculate the ratio
between the sound pressure in the powerhouse, and the sound threshold
pressure for a healthy person.
5. Case
History C
A 9-year-old girl suffers from
facultative hypermetropia. She is placed 6 m from the Snellen test chart and
asked to read line 6, which is the letter size read by a normotropic eye. When
thin convex lenses are placed in front of her eyes, she can still read line 6.
The diopter of the strongest convex lens with which she can still read line 6 is
+5 D for both eyes. Examination with concave lenses reveals the strongest
concave lens by which she can read line 6 to be -4 D.
-
Where are the far point (F) and
the near point (N) located?
-
Calculate her accommodative power
and compare it to the normal value of 14 D.
-
Calculate the correction needed.
5. Case
History D
A 40 year old male diabetic has an
accommodative power of 10 D. His near point (N) is located 0.05 m in front of
the eye (- 0.05 m).
-
1. Calculate the location of F and
the necessary correction.
-
2. What is the name of the
refractive disorder?
-
3. Is the patient capable of driving
a car without corrective glasses? Is the vision mildly or seriously reduced?
Try to solve the problems before
looking up the answers.
Highlights
-
Near vision- even with only one eye
- triggers accommodation and pupillary constriction.
-
Rods are most sensitive in the dark
(scotopic vision). More than hundred rods converge on each ganglion cell.
There are no rods at all in the fovea.
-
Cones operate best in light (photopic
vision). Cones have a high-resolution capacity and hence a high visual acuity,
because the light is focused on the fovea, where the cones are concentrated.
-
The cornea has a refractive power
of 43 D, and the healthy lens varies between 12 and 26 D.
-
The accommodative power of the eye
is the rise in refractive power from the un-accomodated to the maximally
accommodated condition.
-
Conjugate movements are movements
of both eyes in the same direction and magnitude, so that the relation between
the visual axes is maintained.
-
Saccadian or jumping movements are
rapid eye movements. Saccadian eye movement is an instantaneous reposition of
the eye that occurs. when reading or when focusing on a flash of light in the
peripheral visual field. The velocity of the movement is up to 500o per s. The latency period is 250 ms, and the contraction time is 50 ms. The
compensatory eye movement involving the vestibular system, occurs when the
head rotates. This is also an example of Saccadian eye movement.
-
The fovea only contains cones.
Cones function in the daytime, with maximal visual acuity, and colour vision.
The human eye possesses three types of cones, each with a specific pigment
related to the three basic colours: red (erythrolab), green (chlorolab) and
blue (cyanolab).
-
The 3 cone types are uniformly
distributed in the retina except in the fovea. The fovea has no cyanolab cones
and no rods.
-
Abnormal trichromats have a reduced
amount of one cone pigment: Protanomalous trichromats lack erythrolab,
deuteranomalous (the most frequent type) lack chlorolab, and tritanomalous
lack cyanolab.
-
Movements in the visual scenery are
depicted as opposite movements on the retina. Convergent inputs from the two
eyes result in depth perception (ie, stereopsis or stereoscopic vision).
Stereopsis depends upon the medial, longitudinal fasciculus and the corpus
callosum. These structures co-ordinate the movements of the two eyes.
-
A flickering source of light
liberates successive flashes so rapidly that they fuse and appear continuous.
-
The normal human ear is sensitive
to pure tones with frequencies between 10 and 30 000 Hz in a young person.
-
Speech has an intensity of 60-65 dB
and sounds that exceed 100 dB can damage the ear.
-
A high frequency tone produces
travelling waves along the basilar membrane. High tones travel only a short
distance from the stapes along the basilar membrane to their resonant point,
where the displacement amplitude of the basilar membrane is maximal.
-
Low frequency tones travel all the
way to the apex of the cochlea. The higher the tone frequency, the more basal
located in the cochlea is the resonant point and its potential.
-
The auditory and the vestibular
systems share the labyrinth, and transmit signals to the brain through the 8th cranial nerve. The two systems record fluid movements and use the so-called
hair cells as mechanical receptors.
-
The medial geniculate nucleus and
the inferior collicle can increase its tone selectivity by dampening other
sound signals. This explains the cocktail party effect.
-
The cupula is concerned with
equilibrium control during motion and with angular acceleration (rotation of
the head).
-
The utricles and saccules are
sensitive to linear acceleration.
Further
Reading
Berardi, N., A. Cellerino, L.
Dominici, M. Fagiolini, T. Pizzorusso, A. Cattaneo, and L. Maffei.
"Monoclonal antibodies to nerve growth factor affect the postnatal
development of the visual system." Proc. Natl. Sci. , USA, 91 (2):
684-688, 1994.
Stryer, L. "Cyclic GMP cascade
of vision." Annual Rev. Neuroscience 9: 87, 1986.
Brodal, A. "Neurological anatomy
in relation to clinical medicine." Edition 3. New York, 1981, Oxford
University Press.
Von Bekesy, G. "Experiments in
hearing." New York, 1960. Mc-Graw-Hill.
Return
to content
Return
to chapter 5
|