Study
Objectives
· To define asystolia, atrial fibrillation, atrial flutter,
axis-deviation, bradycardia, heart block, tachycardia, sinus rhythm,
ventricular fibrillation, ventricular tachycardia and vulnerable period.· To describe cells with pacemaker-function, the normal impulse
conduction in the heart, the recording of the electrocardiogram (ECG), and
different types of heart block. ·
To draw membrane potentials of sinus cells, atrial cells, and
myocardial cells during a cardiac cycle, and draw a normal ECG. ·
To estimate the electrical QRS-axis from the R-waves of the standard
limb leads. ·
To explain the effect of the autonomic nervous system on the membrane
potential of the sinus node. To explain the genesis of the ECG in health and disease. To explain the Adam-Stokes syndrome. ·
To use these concepts in problem solving and case histories.
Principles
The
body conducts electrical signals uniformly in all directions (an almost
uniform volume conductor increasing the potential electrical field around the
heart generator). ·
Einthoven’s law states that any two of the three bipolar standard limb
leads determine the third one with mathematical precision.
Definitions
· An electrocardiogram (ECG) is a curve showing the potential variations against
time in the whole body stemming from the heart, which is an electrochemical
generator suspended in a conductive medium.
· Asystolia refers to cardiac arrest.
· Atrial fibrillation is a continuous atrial activation with 400 or more
contractions per min. Contractions spread through the atrial tissue almost
without mechanical effect and only few electrical signals are conducted to the
ventricles.
Atrial
flutter is an atrial contraction rate around 300 per min, often with every
second contraction conducted to the ventricles. Sawtooth-like flutter waves
characterise the ECG.
· Bradycardia is an unduly slow heart rate. Sinus
bradycardia is a sinus
rhythm at rest below 60 beats per min during the day or less than 50 at night.
· Calcium concentration in plasma (total): Normal range is 2-2.5 mM.
· Heart block is a blockage somewhere along the pathway for impulse conduction in the
heart.
· Mean QRS- axis of the ventricles or the mean
cardiac vector is the net force in the frontal plane during ventricular
depolarisation and repolarisation. Many electrical potentials are propagated
in different directions and most of these cancel each other out. The main
direction of the mean cardiac vector is from the base of the ventricles
towards the apex.
· Left-sided axis deviation is characterised by a positive RI and a negative RIII (Fig.
11-7). Cardiologists use the net area of the QRS-complex for precise
diagnosis.
· Pacemaker cells are small pale cells located in the sinus node of the heart. The sinus
node is the primary determinator of the cardiac rhythm, because its cells have
the highest spontaneous frequency.
· Potassium concentration in plasma: Normal range is 3.5-5 mM.
· Right-sided axis deviation is characterised by a negative RI and
a positive RIII (Fig.
11-7). Cardiologists use the net area of the QRS-complex for precise
diagnosis.
· Sinus rhythm refers to the normal cardiac pacemaker rhythm from the sinus node. The
spontaneous discharge at rest is usually 100 beats per min, but the
parasympathetic inhibitory tone predominates in healthy individuals resulting
in a resting heart rate around 75 beats per min.
· Tachycardia refers to a cardiac rate above 100 beats per min. Sinus tachycardia is a sinus rhythm above 100, which can be caused
by anaemia, cardiac failure, catecholamines, emotion, exercise, fever,
pregnancy, pulmonary embolism or thyrotoxicosis.
· Ventricular tachycardia is defined as three or more ventricular beats
occurring at a rate of 120 beats per min or more.
· Ventricular fibrillation is an extremely rapid ventricular activation without
pumping effect. Electrical defibrillation is the only effective therapy.
· Vulnerable period is a dangerous period in cardiac cycle just at the end of the
contraction (simultaneous with the T-wave in the ECG). Electrical conversion
(an electrical shock) given during this period may in itself initiate
ventricular fibrillation. Refractory areas of cardiac muscle are spread among
non-refractory areas.
Essentials
This
paragraph deals with 1. Neural
regulation of the heart function, 2. The action
potential, 3. The role of calcium, 4. Spontaneous
depolarisation, 5. Development of
electrocardiography,
and 6. The normal ECG.
1.
Neural regulation of the heart function
A
large number of sympathetic (S) and vagal (X) motor nerve fibres end close to
the sinoatrial node (SA in Fig. 11-1).
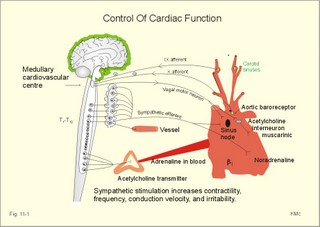
Fig. 11-1: The neural control of the heart.
Sympathetic
stimulation speeds up the sinus node (sinus
tachycardia) and vagal activity slows the node (sinus bradycardia). Increased concentration of the sympathetic
transmitter noradrenaline, and of adrenaline from the adrenal glands, cause positive
inotropic state (increased contractility), positive
chronotropic state (increased frequency),
positive dromotropic state (increased conduction velocity), and positive bathmotropic state (increased irritability) on the heart.
Noradrenaline activates a-adrenergic constrictor receptors in the coronary vessels, whereas
adrenaline activates b-adrenergic vasodilatator receptors.
The
neurotransmitter acetylcholine, activating muscarinic receptors, and vagal
stimulation causes reduced contractility (negative inotropy), reduced
frequency (negative chronotropy), reduced conduction velocity (negative
dromotropy), and reduced irritability (negative
bathmotropy).
2.
The action potential
Across
the ventricular cell membrane there is a steady potential difference of almost
the same size as the equilibrium potential for K+ (-94 mV), that is -90 mV (Fig. 11-2). This negative potential is referred to as the resting
membrane potential (RMP),
because it represents the potential difference across the cell membrane
(inside negative) at rest between successive action potentials.
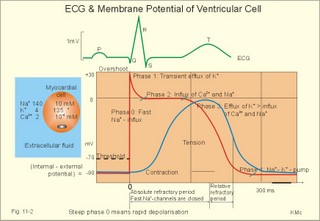
Fig.
11-2: Recordings of ECG (above), intracellular membrane potential (red
curve) and contraction (blue curve) of one heart cycle in a ventricular fibre.
Any
process that reduce the absolute size of the RMP (ie, depolarise the membrane)
tends to activate (open) fast Na+-channels. These
channels contain fast opening and fast closing gates (inactivation gates).
Electrochemical forces favour the abrupt influx of Na+ from
neighbouring regions. Hereby, the potential is further diminished and more and
more Na+-channels are activated or opened. The threshold potential
for release of an action potential is a rise of 25 mV from -90 mV. The cardiac
action potential is an all-or-none
response, which can be divided into five
phases:
The fast
depolarization (phase
0) is shown by the abrupt upstroke, which is related to the rapid entry of Na+ into the cell through the fast Na+-channels,
suddenly allowing the electrostatic and chemical forces to work (Fig. 11-2).
The fast Na+-influx
causes phase 0 of atrial, ventricular and Purkinje action potentials (Fig.
11-2). The fast Na+-channels are both voltage-
and time-dependent. Phase 0 stops at
about +30 mV, because the fast Na+-channels become
voltage-inactivated by closure of inactivation gates. The potential difference
approaches the equilibrium potential for Na+ (+ 60 mV), but only
reaches +30 mV. The conduction velocity along the fast response fibre
increases with the AP-amplitude and especially with the slope of phase 0.
Phase
1 is the
early repolarization from the upstroke. This is related to K+-outflux.
Phase 2 is the plateau of the action potential, where the slow Ca2+-Na+-channels remain open for a long period - up
to 300 ms. The net influx of Ca2+ and Na+ is almost
balanced by a net efflux of K+, so the balance is forming the plateau (Fig. 11-2). Ca2+ activates the muscle contractile process. When the slow Ca2+-Na+-channels
close at the end of the plateau, the voltage-gated K+-channels are
activated, and the permeability for K+ increases rapidly.
Phase 3 is the terminal repolarization. With all the K+-channels open, large amounts of K+ diffuse out of
the ventricular fibre. The equilibrium potential for K+ (-94 mV)
and the RMP is rapidly approached.
Phase 4 is recognized by the RMP of - 90 mV (Fig. 11-2). The Na+-K+ pump restores ionic concentrations by exchanging Na+ for K+ in a ratio of 3:2.
Phase
5 covers the relative refractory
period (RR), and the T-wave in the ECG. The long absolute refractory period (AR) of the ventricular cells covers the whole
shortening phase of the contraction (Fig. 11-2 blue
curve). In the absolute
refractory period all fast Na+-channels are voltage-inactivated and
closed, which prevents sustained tetanus. As a consequence, no stimulus is
sufficient to trigger contraction regardless of size.
In
the relative refractory period, enough of the fast Na+-channels are
recovered, so that a sufficiently large stimulus can break through and produce
an action potential although smaller than normal.
The
long absolute refractory period protects the cardiac pump, as it is not
possible to bring ventricles into smooth tetanus.
As
described above the cardiac muscle fibre has a horizontal plateau, because of
the slow Ca2+-Na+-channels
(phase 2). - Skeletal muscle fibres have no plateau, because they do not open
slow Ca2+-Na+-channels for such a long time.
3.
The role of Ca2+
Cardiac
fibres contain many mitochondria and intercalated desks with gap junctions,
transverse tubules of invaginated sarcolemma, and sarcoplasmic reticulum (Fig.
11-3). The fibres require a continuous supply of oxygen and they are provided
with a rich capillary supply, about one capillary for each cardiac fibre.
The
spontaneous firing from the pacemaker spreads over the entire heart as a
propagating wave.
The
wave of excitation is conducted rapidly along the long axis of a cardiac
fibre, and spreads along the myocardial sarcolemma from cell to cell via
electrically conducting gap junctions.
The
excitation also reaches the interior of the cell through the large T-tubules
filled with mucopolysaccharides. During the phase 2 plateau of the action
potential, Ca2+ permeability increases, and Ca2+ flows
down its electrochemical gradient into the cell through the slow Ca2+-channels.
The channel proteins are phosphorylated by a cAMP-dependent
protein kinase A. The small Ca2+ influx is called trigger-Ca2+,
because it releases large amounts of Ca2+ from the sarcoplasmic
reticulum (Fig. 11-3). Hence the cytoplasmic [Ca2+] increases from
the resting level of 10-7 molar by a factor of 10-100 during
excitation. The free Ca2+ binds to troponin C, just as in striated
muscle cells, and the complex interacts with tropomyosin to activate sites
between the actin and the myosin filaments. This process starts crossbridge
cycling and thus contraction
of the myofibrils.
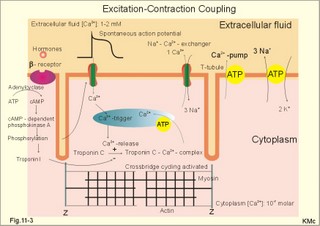
Fig.
11-3: Excitation-contraction coupling in a cardiac fibre.
When
the Ca2+-influx ceases at the end of systole, the Ca2+ -movement is reversed. Now, Ca2+ is pumped into the sarcoplasmic reticulum by a Ca2+ -pump.
The binding of Ca2+ to troponin
C is inhibited by phosphorylation of troponin I, and the binding sites
between actin and myosin are blocked resulting in diastole. In diastole the Ca2+ surplus is removed by a 3 Na+- 1 Ca2+-exchanger, and by
an electrogenic Ca2+-pump (Fig. 11-3).
Catecholamines
and increasing extracellular [Ca2+], raise the cytoplasmic [Ca2+]
and thus the developed force of contraction. This is accomplished in the
following way. Adrenaline and noradrenaline activate adenylcyclase, whereby
cAMP is formed and the dependent proteinkinase
A phosphorylates and activates Ca2+-channel proteins (Fig.
11-3). Hereby, the cytoplasmic Ca2+ is increased, and thus the
force of contraction.
The
excitability of cardiac fibres, striated muscle cells and neurons is reduced
by a reduction of the cellular Ca2+-gradient (hypocalcaemia), a rise in the Na+-gradient across the cell membrane, or the administration of Ca2+-blockers that prevent Ca2+ from entering the
cell.
Cardiac
glycosides, such as digoxin, are beneficial for patients with chronic cardiac
failure. Digoxin inhibits and reduces the number of Na+-K+-pumps
in the membranes of cardiac cells, hereby producing high intracellular levels
of Na+. Some of the intracellular Na+ is exchanged for
extracellular Ca2+, and cytoplasmic Ca2+ enhances the
force of cardiac contraction (positive inotropic effect). Exactly the
necessary minimum dose of digoxin must be used for each patient with cardiac
failure.
In
myocardial cells, as in nerve and skeletal muscle cells, K+ plays a
major role in determining the RMP. At
physiologic concentrations of K+ outside the myocardial cell ([K+]o about 4 mM), the RMP is determined by a dynamic balance between the membrane
conductance to K+ and
to Na+. As [K+]o is increased
(hyperkalaemia), the membrane depolarises. Depolarisation inactivates
voltage-dependent K+ -channels and activates Na+ -channels, allowing Na+ to make a proportionally larger
contribution to the RMP. Increased [K+] in the extracellular fluid
reduces the force of contraction, so the heart becomes dilatated and flaccid.
Because
the equilibrium potential for Na+ is positive (+ 60 mV), it tends
to depolarise the RMP. Since RMP is -90 mV at normal [K+], and the
equilibrium potential of K+ is always more negative (-94 mV), there
is a small outflux of K+ from the cell at equilibrium. The K+ and Na+ gradients are maintained by the efficient Na+-K+-pump.
4.
Spontaneous depolarisation
The
rhythm of the heart is initiated by a complex flow of electrical signals,
which are called action potentials.
The action potentials generated in the sinus node (SN) display automaticity (ie, they undergo spontaneous and rhythmic depolarization without external
stimuli). The sinus node is the primary
pacemaker, because it has the highest frequency. The automaticity (Fig.
11-4) is associated with small pale
round cells in the SN.
The
SN and the AV-node also contain elongated cells that react with a special AP,
a so-called slow response. The AP is
smaller than the fast response, phase 0 is long and caused by slow Ca2+-influx,
phase 1 is absent, phase 2 is slow repolarisation, and the real repolarisation
in phase 3 is due to inactivation of the slow Ca2+-channels and
increased K+–outflux. Phase 4 is horizontal, and the relative
refractory period is long, extending well into phase 4. The slow response
propagates slowly and tends to be blocked, which causes cardiac rhythm
disturbances.
The membrane potential of pacemaker cells (about -55 to -60 mV) is never constant (Fig. 11-4). The diastolic depolarization of pacemaker cells
(producing the very special slope in phase 4 of these cells) is ascribed to an
influx of Na+ through special channels in their cell membrane.
Three or more ionic currents mediate the slow diastolic depolarization: an
inward sodium current (i-funny), an inward calcium current and an outward
potassium current. Towards the end of phase 4 there is also a certain influx of Ca2+ through voltage-activated Ca+2 -channels. Hypocalcaemia diminishes
the amplitude of the action potential and the slope of the pacemaker
potential. The diastolic pacemaker depolarization is opposed by the K+-outflux.
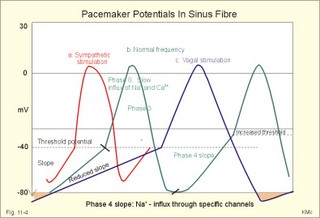
Fig.
11-4: Pacemaker potentials from a sinus nodal fibre: a: Sympathetic
stimulation; b. Normal heart rate; c. Vagal stimulation. There is a constant Na+-influx between two heartbeats. Reduction in the slope of the pacemaker
potential and increase of the threshold both diminish the cardiac frequency.
The
K+-channels remain open during the repolarization, so the membrane
potential approaches -60 mV at the end of the action potential. The rising
pacemaker potential between two heartbeats, is caused by the inherent leaks of
the pacemaker membrane to Na+. The constant Na+-influx causes
the membrane potential to approach the threshold potential. When the membrane potential reaches the threshold potential (about -40
mV), the slow Ca2+-Na+-channels open and the cycle
starts again. This process continues for a lifetime (Fig.
11-4).
The
heartbeat is self-initiating, and normally the propagating wave (impulse)
originates in the sinus node (see above). The impulse propagates from the
sinus node via three bundles of
internodal syncytial cells, through the left and right atrial wall to the atrioventricular (AV) node.
This point is typically reached within 40 ms. After passing through the AV
node (with a so-called AV- delay of 100 ms), the
propagating wave (excitation) reaches the bundle
of His. Here, specialised conduction fibres activate almost synchronously
all the ventricular tissue and thus impart maximal thrust to the blood. The
propagation velocity in these large Purkinje
fibres is 1-4 m per s, which is the fastest velocity possible in the
heart. The Purkinje system terminates just under the endocardial surface on gap junctions in the myocardial cells. The AV-delay provides time
for the atrial systole to pass extra blood to the ventricles before the
ventricular systole occurs.
Adrenergic
transmitters and sympathetic stimulation increase the slope of the diastolic
pacemaker depolarization. Acetylcholine and vagal stimulation increase the K+-efflux,
so the slope is reduced and thus the cardiac frequency is reduced.
Some
cardiac fibres show a slow response comparable to that of the pacemaker cells,
but with a constant phase 4. Ischaemia may activate ectopic pacemaker cells.
5.
Development of electrocardiography
In
1903 Einthoven began a systematic study, with a string galvanometer of the
potential differences between electrodes placed on the skin surface during
heart beats. The string galvanometer was developed to become the first
electrocardiograph. The fluids of the body conduct electricity quite easily.
The body conducts electrical signals uniformly in all directions.
An
electrocardiogram (ECG) is a curve showing the potential variations in time in
the body stemming from the heart,
which is an electrochemical generator suspended
in a conductive medium acting as a volume conductor. The myocardial cell
membranes have separate charges, and small ion gradients produce
electrochemical gradients. Small ion fluxes across the cell membrane only
occur during depolarization and repolarization, where potential differences
are produced between polarised and depolarised tissue regions in the heart.
Each cardiac fibre behaves as a dipole, the magnitude and direction of which
is symbolised by an arrow or a vector. The dipole vector points from minus to
plus by definition.
Originally
Einthoven assumed that the sum of all electrical activity in the heart
resulted in an electromotive force - a main
cardiac vector originating in the middle of the heart.
Einthoven
used three standard bipolar limb leads forming a triangle in the frontal plane
(Einthoven’s triangle in Fig. 11-5 and 11-7). Lead I records the potential difference between the right and left
arms, lead II between the right arm and left leg; and lead III between the
left arm and left leg. The right leg is used to ground the patient (Fig. 11-7;
observe the positive and negative signs). The connections were arbitrarily
chosen so most healthy individuals had dominating upright (positive)
QRS-complexes and T-waves in their leads.
The
actual recording sites are at the junctions between limbs and trunk, because
the arms and legs act as extended
electrodes. Einthoven actually placed the limbs of the patient in bathing
tubes and used these as the first electrodes. Einthoven arranged the ECG
equipment so that the direction of the mean QRS-axis towards the positive pole of a
bipolar lead produces an upright deflection (positive R-waves in the 3 leads shown in Fig.
11-5). When directed
towards the negative pole a downward deflection (a negative RIII-wave in
left-sided axis deviation, Fig. 11-7) was recorded.
Einthoven's law states that any two of the three bipolar limb leads determine the third one
with mathematical precision. The potential differences recorded over time in
an ECG can be estimated using the rules of vectorial projection with force parallelograms in the frontal plane (Fig. 11-5).
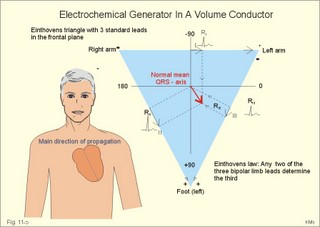
Fig. 11-5: Einthoven's triangle. To the left is
shown the main direction of the conduction system in the frontal plane.
The
R-waves (and QRS deflections) in two of the three standard leads are drawn
graphically in Einthoven’s triangle and their resultant is the mean QRS-axis of the heart or more exact the mean ventricular axis
in the frontal plane (Fig. 11-5). This is a mathematical concept representing
the integral cardiac vector operating in Einthovens triangle.
The mean QRS-axis of the
heart is usually located around 60 degrees in Einthoven’s triangle.
Right- sided
axis deviation is found in children or high thin individuals with a vertical located heart,
and in persons with right ventricular hypertrophy. The axis is now located to
the right and the condition is diagnosed by a positive RIII combined
with a negative RI (between +90 to +180 and even +90 to –90 degrees in Fig.
11-7).
Left-sided axis
deviation is
found with left ventricular hypertrophy, in fat individuals and in late
pregnancy, when the heart is pressed upwards to the left. The condition is
diagnosed by a negative RIII combined with a positive RI (Fig. 11-7).- Actually cardiologists operate with the net
areas of the QRS-complexes in order to diagnose significant deviations.
6.
The normal ECG
The
ECG is recorded from the surface of the body, and used to demonstrate the
presence of AV-blocks, ectopic foci, premature beats, sinoatrial arhythmias,
atrial fibrillation and ventricular fibrillation etc.
Each
heart cycle has a fixed pattern of ECG waves (P,Q,R,S and T). The sinus node
is a minimal muscle mass, and there is no potential difference (wave in the
ECG) before the atria depolarise with a P-wave. When the propagating impulse
wave is directed towards the positive electrode
(as in lead II) the atrial depolarization will produce a positive P-wave (Fig. 11-6). The P-waves correspond to the impulse
distribution in the atria. Retrograde excitation of the atria creates a negative P-wave. When atrial excitation coincides with the QRS, the P-wave is often
hidden. The PR-interval (normally 0.12-0.2 s) measures the impulse speed
through the supraventricular tissue from the sinus node to the bifurcation of
the Hiss bundle. Prolonged PR-interval is caused by disturbances of AV
conduction. The QRS-complex measures the passage through the left and right
bundle branch followed by depolarization of the strong ventricular myocardium
(Fig. 11-6). The QRS-complex is prolonged (> 0.12 s), when the left or
right bundle branch is blocked by disease. When excitation originates in the
ventricles, it spreads slowly and the QRS-complex is severely deformed.
Since
the activation of the septum is mainly from left towards right, the
propagating wave moves away from the exploring electrode, and the Q-wave becomes negative, whereas the dominating ventricular transfer of the
propagating wave towards the apical electrodes provides the large, positive R-wave in almost all leads. The small propagating wave moving away
from the electrode at the apex and to the right to reach the thin-walled right
ventricle, is responsible for the small, negative S-wave. The T-wave represents ventricular repolarization and has the same
direction as the QRS-complex in most normal leads. When the QRS-complex is
positive and the T-wave is negative, it indicates that the repolarization
proceeds in a wrong direction. Abnormal T-waves are due to cardiac
hypertrophy, myocardial damage or electrical disturbances.
The
isoelectric ST-segment represents the period, where the entire ventricular
myocardium is depolarised (therefore isoelectric). Any deviation (up or down
from the isoelectric level) indicates anoxic
damage of the myocardium.
The QRS- plus ST-intervals correspond to the duration of
the ventricular systole.
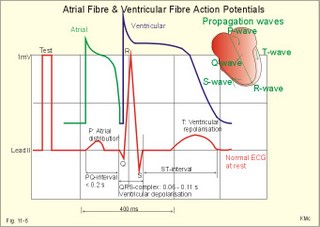
Fig.
11-6: Normal ECG (II. lead). The action potentials from an atrial (green
curve) and a ventricular fibre (blue curve) are shown above. – To the right
is shown the direction of propagating waves in the frontal plane and their
relation to the ECG waves.
The T-wave is positive in most leads, and due to the apical directed
repolarization of the ventricular action potential (Fig. 11-6).
Unipolar,
precordial leads (Wilson) have one exploring
electrode as the actual recording electrode, detecting changes in the local
potential relative to zero. The exploring electrode is defined relative to the
three standard extremity leads, which are connected to form one indifferent reference
electrode. Conventionally, the 6
Wilson leads are recorded from specific locations on the precordium (V1 to V6).
The
exploring electrodes of leads V1 to V3 are located to
the right and are looking at the right side of the heart. The QRS complexes of
the normal heart are mainly negative in these leads. The exploring electrodes
of leads V4 to V6 are located to the left and are looking at the left side of the heart, where
the QRS complexes are typically positive.
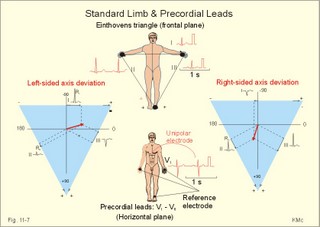
Fig. 11-7: Standard limb leads (Einthoven’s triangle) and precordial
ECG leads.
In unipolar recordings, upright deflection indicates movement of the propagating wave towards the
positive, exploring electrode, and downward deflection (a negative ECG wave)
indicates that the propagation wave is moving away (Fig. 11-7).
Pathophysiology
This
paragraph deals with cardiac
arrhythmias.
Arrhythmia is any cardiac rhythm different from the normal sinus rhythm. Sinus rhythm is
the automaticity elicited from the normal cardiac pacemaker, the sinus node.
The sinus node depolarises spontaneously with a frequency between 60 and 100
beats per min (bpm) at rest. Normally the parasympathetic tone predominates,
whereby the heart rate is reduced from 100 to 60-70 bpm at rest. Any reduction
in parasympathetic tone or increase in sympathetic tone results in tachycardia
(ie, a ventricular pumping frequency above 100 bpm). Reduction in sympathetic
tone or increase of the parasympathetic tone leads to bradycardia (ie, a
ventricular pumping rate below 60 bpm).
Cardiac
arrhythmias are divided into two groups: I. Pacemaker abnormalities, and II. Conduction
abnormalities (cardiac block).
I.
Pacemaker abnormalities
arise
in the sinus node (ie, sinus tachycardia, sinus bradycardia or sinus
arrhythmia) or outside the node (ie, ectopic beats, tachycardia, and
fibrillation and shifting pacemaker). These arrhythmias are disorders of
rhythmogenesis.
Sinus node
disease is
a mixture of conditions with insufficient discharge of signals from the sinus
node. The sick sinus syndrome is
caused by damage of the nodal tissue. Sinus pauses lead to sinus bradycardia,
tachycardia, tachy-brady-cardia syndrome, ectopic beats or atrial arrest
(sinus arrest).
Sinus
tachycardia (heart
rate above 100 bpm) is caused by psychological (panic, anxiety) or physical
stress (anaemia, hypoxia, exercise, fever, intoxication, shock etc). Any
condition with increased sympathetic tone results in tachycardia. Adrenergic b-blockers are effective in slowing down the
heart rate.
Sinus
bradycardia (heart rate below 60 bpm) is a normal phenomenon in well-trained people and in
elderly persons. Athletes at rest have a high stroke volume and a low pulse, because they are dominated by vagal tone in this condition. All
persons react with sinus bradycardia, during hypothermia, hypothyroidism, jaundice, increased intracranial pressure, treatment with digoxin or b-blockers, and in sinus node disease.
Sinus
arrhythmia is
a normal phenomenon. There is a rise in heart rate during inspiration followed
by a fall during expiration. During inspiration, the low intrathoracic
pressure improves the venous return, which – with a delay through the
pulmonary circulation - increases the stroke volume of the left ventricle. The
resulting increase in aortic intravascular pressure combined with the low
intrathoracic pressure around the aorta leads to an increased transmural
pressure over the aortic wall and thus to a strong stimulation of the aortic
baroreceptors. This is why the vagal tone falls and the sinus node discharge
quickens. The reflex and circulation delay explains why the fall in heart rate
manifests itself during expiration and not during inspiration.
Ectopic beats originate in pacemaker cells outside the sinus node. The abnormal pacemaker
tissue is triggered by ischaemia, mechanical or chemical stimuli. Cardiac
catheterisation can trigger ectopic beats. Ectopic beats are either of atrial
or ventricular origin.
1)
Atrial ectopic beats appear as early (premature extrasystoles) and abnormal P-waves in the ECG;
they are usually followed by normal QRS-complexes (Fig.
11-8). Following the
premature beat there is often a compensatory interval. A premature beat in the
left ventricle is weak because of inadequate venous return, but after the long
compensatory interval, the post-extrasystolic contraction (following a long
venous return period) is strong due the Starling´s law of the heart. -
Adrenergic b-blockers are sometimes necessary.
2)
Ventricular ectopic beats (extrasystoles) are recognized in the ECG by their wide QRS-complex (above
0.12 s), since they originate in the ventricular tissue and slowly spread throughout the two ventricles without passing the Purkinje
system. The ventricular ectopic
beat is recognized by a double R-wave (Fig. 11-8). The classical tradition of
simultaneous cardiac auscultation and radial artery pulse palpation eases the
diagnosis. Now and then a pulsation is not felt, and an early frustraneous
beat is heard together with a prolonged interval. A beat initiated in the
vulnerable period may release lethal ventricular tachycardia, since the tissue
is no longer refractory.
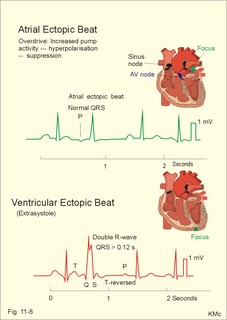
Fig.
11-8: Atrial (left) and ventricular (right) ectopic beats.
After
a period with high cardiac frequency from activity of an ectopic focus (ie,
overdrive), there often follows a period with a remarkable fall in frequency
(ie, overdrive suppression). During the overdrive, the Na+-K+-pump
is extremely active in order to extrude Na+ from the myocardial
cells in the short phase 4 periods, and the Na-influx exceeds the K-influx
(Na:K= 3:2). Hereby, the cell becomes hyperpolarised in the end, which may
stop the high frequency and turn it into suppression.
Tachycardia occurs in paroxysms and is either of
atrial or ventricular origin.
1) Atrial tachycardia is elicited in the atrial tissue outside the SN as an
atrial frequency around 200 bpm. Often only every second impulse passes the
AV-node to the ventricles, so a 2.1 AV-block is found in the ECG (Fig. 11-9).
2) Ventricular tachycardia is elicited from one focus in the ventricular tissue
with a frequency around 200 bpm (more than 120 bpm) and abnormal
intraventricular impulse conduction (disturbed QRS complexes). Of course,
there are no P-waves in the ECG, and the QRS-complexes are broad and irregular
(Fig. 11-9).
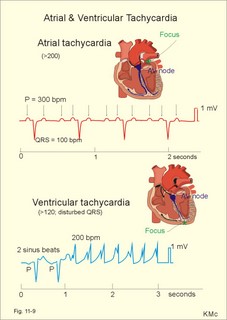
Fig.
11-9: Left: Atrial tachycardia with a QRS-frequency of 100 bpm. - Right:
Ventricular tachycardia with a QRS-frequency of 200 bpm following 2 sinus
beats.
Fibrillation
is either atrial or ventricular in origin.
1)
Atrial fibrillation is a condition in which the sinus node no longer controls the rhythm and the
atrial muscle fibres undergo a tumultuous rapid twitching. A total
irregularity of ventricular contractions characterise the fibrillation. An
excitation wave with 400-600 cycles per min, courses continuously through the
atrial wall over a circular pathway about the origin of the great veins (the circus
motion theory). There is a continuous activation with more than 400
P-waves per min, where regular atrial contraction is impossible. It is difficult to see and count the P-waves of the ECG. Because of the
refractoriness of the AV-bundle, only some of the excitation waves result in
ventricular beats. The pulse of the patient is therefore irregular as the
occurrence of QRS-complexes in the ECG. The many P-waves (also called f-waves
for fluctuations) are characteristic for atrial fibrillation. Untreated atrial
fibrillation has a QRS-frequency of 150-180 bpm (Fig.
11-10). Old patients
with chronic heart disease often show the so-called slow
atrial fibrillation with a QRS-frequency below 60 bpm.
Most cardiac disorders can lead to atrial fibrillation or
flutter.
Atrial flutter is related to atrial fibrillation, but the atrial frequency - counted from the
P-waves - is much lower than 400 bpm - usually around 300 bpm and the
AV-conduction is more regular. The consequences to the patient depend upon the
number of impulses conducted from the atria through the AV-node to the
ventricles (recorded as QRS-complexes). Often every second impulse reaches the
ventricles, so the ratio of AV-blocks is 2:1, but the ratio can also be 3:1,
4:1 etc. Atrial flutter is recognized in the ECG as sawtooth-like P-waves
(Fig. 11- 10).
2)
Ventricular fibrillation is a tumultuous twitching of ventricular muscle
fibres, which are ineffectual in expelling blood. The condition is lethal
without effective resuscitation. The irregular ventricular rate is 200-600
twitches/min. Without contractile co-ordination the force is used
frustraneous. Actually, the heart does not pump blood, so within 5 s
unconsciousness occurs, because of lack of blood to the brain. In patients
with coronary artery disease, ventricular fibrillation is a cause of sudden
death. The trigger is anoxia (with
an ineffective Na+-K+-pump) and the impulses arise from several foci in the ventricular
tissue. There is no regular pattern in the ECG. Ventricular fibrillation is
initiated when a premature signal arrives during the downslope of the T-wave (vulnerable
period). Electrical shock (electrocution) also triggers ventricular
fibrillation.
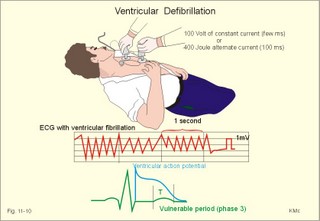
Fig. 11-10: Ventricular
defibrillation of a patient with cardiac arrest.
Ventricular
fibrillation is the most serious cardiac arrhythmia. It must be converted to
sinus rhythm at once by the application of a large electrical
shock to the heart (ventricular defibrillation) or the patient will die.
Alternating current is applied for 100 ms or 1000 volts direct current is
applied for a few milliseconds. The vulnerable period (VP in Fig. 11-7 is actually phase 3 and represented in the ECG as the T-wave) is
dangerous, because an electrical shock, when given during this period, will
cause in itself ventricular fibrillation.
Here is shown sinus rhythm and one ectopic beat followed
by ventricular fibrillation. The only effective treatment is rapid institution
of electrical defibrillation.
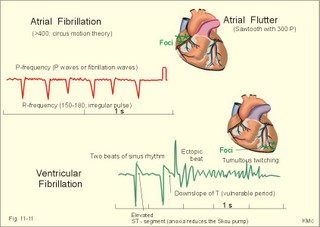
Fig.
11-11: Left: Atrial fibrillation with a QRS-frequency of 180 bpm. - Right:
Ventricular fibrillation following 2 beats of sinus rhythm and one ectopic
beat.
Shifting pacemaker is a condition where the impulse
originates in shifting locations inside the SN, or the pacemaker shifts from
the SN to the AV-node. In the first case the P-wave change size from beat to
beat, and in the second case the P-wave is found either in front of the
QRS-complex or behind.
II.
Conduction abnormalities
are 1) sino-atrial block, 2) atrioventricular
block, 3) bundle branch block, 4) WPW- syndrome, and 5) the long QT-syndrome.
1) Sino-atrial block (see SN disease) is characterized
by long intervals between consecutive P-waves, and caused by blockage of the
formation or conduction of the stimulus from the SN to the atrial tissue
(ischaemia or infarction of the SN).
2) Atrioventricular
block is blockage of the conduction from the atria to the AV-node.
The first-degree AV
block is a prolongation of the PQ (PR)-interval (above 0.2 s) implying a
delay of the conduction - not a real block. All beats are conducted, so there
is a QRS-complex following each P-wave, although with delay (Fig.
11-12).
The
second-degree AV block occurs when some signals are not conducted to the AV-node, so some of
the P-waves are not followed by QRS-complexes. The ventricles actually drop
some beats. A typical example is Mobitz type I block or Wenchebach block,
which is a predictive loss of a QRS-complex. The PQ-interval is increased
progressively until a P-wave is not followed by a QRS-complex. Mobitz type II
block occurs without warning. Suddenly, a QRS- complex falls out (Fig. 11-12).
The third
degree AV block (complete AV-block) is a total block of the conduction between the SN
and the ventricles. Also blocked Hiss bundle conduction results in an AV-block
(Fig. 11-12). An AV- or
ventricular pacemaker maintains life with a spontaneous escape rhythm around 40-50 bpm, or cardiac arrest occurs with the fainting paroxysms of
Adam-Stokes syndrome.
The
Adam-Stokes syndrome is a clinical disorder caused by a partial AV-block, with a long P-Q interval and a wide QRS complex in the ECG,
suddenly becoming a total bundle block. The condition results in
unconsciousness and cramps caused by brain hypoxia and sometimes resulting in
universal cramps (grand mal) due to
violent activity in the motor cortex. The keyhole is the AV node and the
bundle of His. Disease processes here elicit the Adam-Stokes syndrome. Therapy
is to provoke sinus rhythm by a few forceful strokes in the precardial area of
the thorax, accompanied by mouth-to-nose-resuscitation and external heart
massage. A sympathomimetic drug can be injected if necessary even in the heart
directly. The patient must be immediately brought to hospital for special
intensive care. Permanent pacemaker treatment may become necessary.
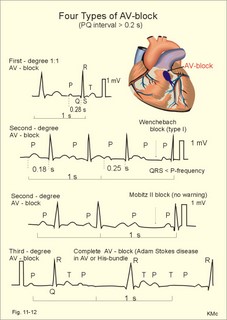
Fig.
11-12: Four types of atrio-ventricular (AV)-block. From above downwards:
First-degree AV-block, Second-degree Mobitz I block (Wenchebach),
Second-degree Mobitz II block, and Complete AV-block.
3) Bundle
branch block is a block of the right or the left bundle branches. The signal is conducted
first through the healthy branch and then it is distributed to the damaged
side. This distribution takes more time than usual, so the QRS-complex is
wider than normal (more than 0.12 s in Fig. 11-13).
In right bundle branch block, the right ventricle is activated late,
which is shown by a tall double R-wave in V1 (ie, the second late R-wave is
from the right side), and a deep wide S-wave in leads I and V6 .
The left bundle branch block is characterized by a late activation of
the left ventricle from apex towards basis. This results in a solid R-wave in
the left precardial leads (V5 andV6), whereas there is a deep broad S-wave in V1 and III (Fig. 11-13).
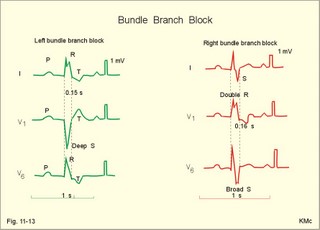
Fig.
11-13: Right and left bundle branch block.
4) WPW-syndrome or Wolf-Parkinson-White block is not
a direct block of the conduction through the Hiss bundle and branches, but is
caused by a short cut through an extra conduction pathway from the atria to
the ventricles. This abnormal conduction pathway is congenital and called the bundle
of Kent (Fig. 11-14). Due to this short-cut, the slow conduction through
the AV-node is bypassed and the ventricles are depolarised faster than normal.
The WPW-syndrome is recognized in most ECG leads as a short PQ (PR)-interval
followed by a wide QRS-complex with a delta wave (Fig. 11-14 ). The patients
often have paroxysmal tachycardia or they may develop atrial fibrillation.
Some
patients are treated with ablation of the bundle of Kent. Other patients are asymptomatic and in good physical
condition.
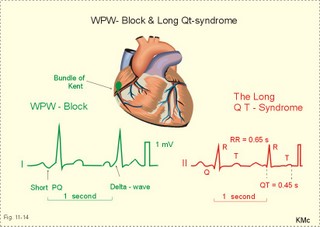
Fig.
11-14: The WPW-syndrome and the long QT-syndrome.
5) The long
QT-syndrome.
This is frequently a genetic condition, where fast repolarised cells are
restimulated by cells that have not repolarised. When acquired the condition
is caused by myocardial ischaemia, by drugs or by a low serum [Ca2+] - below 2 mM. Normally, the QT-interval is less than 50% of the
preceding RR-interval (Fig. 11-14). The long QT-interval symbolises a long
ventricular systole. Actually, the ST-interval is simultaneous with the phase
2 plateau of the ventricular membrane action potential. Here, the slow Ca2+ -Na+ - channels remain open for more than 300 ms as normally. The
net influx of Ca2+ and Na+ is almost balanced by a net
outflux of K+. Hereby, a long phase 2 plateau or isoelectric
segment is formed.
Cardiac
pacemakers
Implanted
cardiac pacemakers are successful in keeping heart patients alive. This is
often a beneficial treatment of Adam Stokes syndrome or ventricular
tachycardia. An electrical pacemaker is a small stimulator with battery
planted underneath the skin. The electrodes are connected to the right
ventricular muscle tissue, whose contraction rate is controlled by the
stimulator.
Cardiopulmonary
resuscitation
Cardiac
arrest is cessation of all spontaneous cardiac rhythmicity. Cardiac arrest is
most often caused by anoxia. The cause of anoxia is inadequate respiration due
to terminal lung disease, thoracic trauma, and shock or deep anaesthesia.
Cardiopulmonary
resuscitation is important in keeping the heart alive until electrical
defibrillation can be performed with a large electrical shock. Alternating
current is applied for 100 ms, or 1000 mV direct current is applied for a few
ms.
Ventricular
hypertrophy
The consequences of the rise in cardiac mass are the same
for the locally recorded ventricular fibre action potentials. The action
potentials increase in magnitude, with a parallel increase of QRS voltage
observed in the ECG. The heart partially adapts to the increase in workload by
an increase in muscle mass (hypertrophy). The degree of hypertrophy is roughly
proportional to the increase in load. As the electrically active surface area
is increased, there is an increase in ventricular fibre action potential, and
thus in the amplitude of the R wave in the left precordial leads.
The ECG criterion of left ventricular hypertrophy is that the sum
amplitude of S in V1 and
R in V6 is larger than 3.5 mV (3.5 cm).
With
a delay in conduction through the large left ventricle - or with left bundle
branch block - there is a wide QRS complex.
The
normal positive T-wave is due to the apical directed repolarization of the
ventricular AP. Therefore, asymmetrical T-inversion or bi-phasic T-waves and
downward-sloping ST-segment signal abnormal repolarization with the
propagating wave moving away from the apical electrode in most leads
(so-called strain pattern in Fig. 11-15).
Left
axis deviation is often found in the standard leads of left ventricular
hypertrophy patients (augmented R-wave in I and S-wave in III).
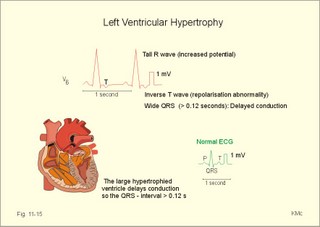
Fig.
11-15: ECG from a patient with left ventricular hypertrophy.
The
disorders causing left ventricular
hypertrophy are:
Heart diseases:
Myocardial disorders, pericarditis, valvular disorders, congenital heart
disease.
Vascular
disorders:
Atherosclerosis, systemic hypertension (Chapter
12) , aortic stenosis, renal
disorders, arteriovenous shunts, and aneurysms.
Thoracic
diseases:
Diseases of the lungs & pleura, and kyphoscoliosis.
Pumping of
increased volume load: Acromegaly, anaemia, obesity and excessive alcohol
intake, thyrotoxicosis, severe manual work and sports.
Left
ventricular hypertrophy is often demonstrated by echocardiography or found on
the ECG.
Self-Assessment
Multiple Choice Questions
I. Each of the following five statements have True/False options:
Stem statement: The ventricular action potential is
A. initiated by rapid entry of Na+.
B. characterised by slow Ca2+ -Na+- channels.
C. characterised by closed K+- channels in phase 3.
D. dependent upon Ca2+-influx.
E. independent of the Na+-K+ -pump in phase 4.
II. Each of the following five statements have True/False options:
A. In myocardial cells, as in nerve and skeletal muscle cells, K+ plays a minor role in determining the resting membrane
potential.
B.
The impulse propagates from the sinus node via five bundles of internodal
syncytial cells through the left and right atrial wall to the atrioventricular
node.
C. The long absolute refractory period of the ventricular cells, covers the whole shortening phase of the contraction, where all the
fast Na+-channels are voltage-inactivated. As a consequence, no
stimulus is sufficient regardless of size.
D. The fast Na+-influx causes phase 0 of atrial- , ventricular- , and
Purkinje- action potentials. The fast Na+-channels are both
voltage- and time-dependent.
E. Noradrenaline
activates a-adrenergic
constrictor receptors in the coronary vessels, whereas adrenaline activates b-adrenergic
vasodilatator receptors.
III. The following five statements have
True/False options.
A. WPW-syndrome or Wolf-Parkinson-White block is caused by a short cut
through an extra conduction pathway from the atria to the ventricles.
B.
Atrial fibrillation is more malignant than ventricular fibrillation.
C. All pacemaker abnormalities arise in the sinus node.
D.
Premature beats are also called atrial ectopic beats.
E.
Only few cardiac arrhythmias can lead to atrial fibrillation and flutter.
Try to solve the problems before looking up
the answers.
Highlights
· The heart rate is controlled mainly by the autonomic nervous system.
Sympathetic stimulation speeds up the sinus node (sinus tachycardia) and vagal
activity slows the node (sinus bradycardia).
· The autonomic nervous system controls myocardial contraction by varying
the Ca2+ -permeability
of the sarcolemma via hormones and the adenylcyclase system.
· Increased concentration of the sympathetic transmitter noradrenaline,
and of adrenaline from the
adrenal glands, cause increased contractility, increased frequency, increased
conduction velocity, and increased irritability of the heart.
· Catecholamines and increasing extracellular [Ca2+], raise the
cytoplasmic [Ca2+] and thus the developed force of contraction.
· Cardiac digitalis glycosides (digoxin) block the Na+-K+-pump.
This blockage increases the internal [Na+] to the extent that less Ca2+ is removed from the
cell. This - and any - form of elevated cytoplasmic [Ca2+] enhances
contractile force. Increased [K+] in the extracellular fluid
reduces the force of contraction, so the heart becomes dilatated and flaccid.
· Noradrenaline activates a-adrenergic constrictor receptors in the coronary vessels, whereas
adrenaline activates b-adrenergic vasodilatator receptors.
· The neurotransmitter acetylcholine, activating muscarinic receptors, and
vagal stimulation cause reduced contractility (negative inotropic state),
reduced frequency (negative chronotropic state), reduced conduction velocity
(negative dromotropic state), and reduced irritability (negative bathmotropic
state).
· Changes in blood concentrations of gasses and protons affect the cardiac
function directly and indirectly via chemoreceptors.
· The long absolute refractory period of the ventricular cells, covers the whole shortening phase of the
contraction, where all the fast Na+-channels are
voltage-inactivated. As a consequence, no stimulus is sufficient regardless of
size.
· The electrocardiogram (ECG) is a surface recording of the electrical
field generated in the entire body by the heart.
· The sinus node is a minimal muscle mass, and there is no potential
difference (wave in the ECG) before the atria depolarise with a P-wave. When
the propagating wave is directed towards the electrode (as in lead II) the
atrial depolarization will produce a positive P-wave.
· The P-waves correspond to the impulse distribution in the atria, and the
QRS-complex origin from depolarisation of the strong ventricular myocardium.
· The QRS deflections in two of the three standard leads can be drawn
graphically in a triangle and their resultant is the mean QRS-axis of the
heart.
· The T-wave is caused by the spread of repolarization over the
ventricles.
· The small propagating wave moving away from the electrode at the apex
and to the right to reach the right ventricle, is responsible for the small,
negative S-wave.
· The Adam-Stokes syndrome is a clinical disorder caused by a partial
AV-block, with a long P-Q interval and a wide QRS complex in the ECG, suddenly
becoming a total bundle block. The condition results in unconsciousness and
cramps caused by brain hypoxia and sometimes resulting in universal cramps
(grand mal) due to violent activity in the motor cortex. Disease processes in
the AV node and the bundle of His elicit the Adam-Stokes syndrome.
· Ventricular tachycardia is defined as three or more ventricular beats
occurring at a rate of 120 beats per min or more.
· Ventricular fibrillation is an extremely rapid ventricular activation
without pumping effect. Electrical defibrillation is the only effective
therapy.
· Vulnerable period is a dangerous period in cardiac cycle represented in
the ECG as the downslope of the T-wave. Electrical conversion (an electrical
shock) given during this period causes in itself ventricular fibrillation.
Further
Reading
Cardiovascular Reviews & Reports. Monthly
journal published by Le Jacq Communications Inc, 777 West Putnam Av.,
Greenwich CT, 06830, USA.
Kastor, JA (2000) Arrhythmias. 2nd Ed. Philadelphia:
W.B.Saunders Co.
Surawicz, B (1995) Electrophysiological
Basis of ECG and Cardiac Arrhythmias. Baltimore: Williams and Wilkins.
Return
to content
Return
to chapter 11
|